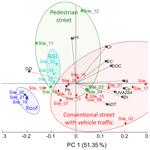
Contaminants in Urban Stormwater: Barcelona case study
Diego Schmidlin
Laura Scheiber
Maria José Chesa
Enric Vázquez-Suñé
Today's cities face simultaneous challenges due to rapidly growing populations, urban sprawl, climate change, and environmental pollution which pose a pressure on our traditional urban drinking water supplies. In this context, stormwater could augment our over-drafted urban groundwater resources. However, urban stormwater runoff carries a myriad of dissolved contaminants (e.g., organics, metals, nutrients), which pose a serious risk to the environmental and public health. Moreover, dissolved contaminants of urban origin – such as trace metals and organic compounds of emerging concern – may not be adequately removed by conventional stormwater treatments. Therefore, it is of the utmost importance to fully understand stormwater contaminant presence, transport, and fate in the built environment to design novel or improve conventional treatment systems. To address this knowledge gap, we have conducted 7 field sampling campaigns during storm events at different Barcelona locations (within 3 districts) to investigate contaminant presence in different urban compartments (e.g., roofs, conventional streets with automobile traffic, pedestrian streets, and green infrastructure outlets). Preliminary results have confirmed presence of toxic metals in Barcelona urban rain and stormwater runoff along with significant differences depending on the catchment areas. After a storm event, trace metal concentrations followed the order: roof rain < pedestrian street runoff < conventional street runoff. Additionally, blue-green infrastructures (bioretention systems) had lower mean metal concentrations at the effluent (outlet) than the influents (inlet). Our initial results on metal occurrence in stormwater collected in the city of Barcelona will provide stormwater quality foundation for water agencies, municipalities, and companies in other water-stressed regions with Mediterranean climate.
- Article
(1835 KB) - Full-text XML
- BibTeX
- EndNote
The availability of water has become one of the biggest challenges of the present century (Liu et al., 2017). This is particularly important in densely populated cities under a Mediterranean climate (e.g., Barcelona, Spain). Water shortages in these regions are becoming more evident in this century due to population growth, economic development, and impacts of climate change (Luthy and Sedlak, 2015). In parallel, cities make a lot of effort to control floods and pollution during storm events. Under this scenario, the capture, treatment, and recharge of urban stormwater runoff could enhance our city water supplies. In fact, several cities (e.g., Barcelona) have started to harvest stormwater, though the main use is still non-potable, e.g., street sweeping or irrigation (Barcelona City Council, 2020).
One of the main barrier to include stormwater as alternative to augment water supplies is that urban stormwater runoff is generally polluted (Björklund et al., 2018). Numerous studies have investigated the presence of contaminants in stormwater, mainly focusing on total suspended solids (TSS), pathogens, nutrients, metals, organic compounds (hydrophobic and hydrophilic), and microplastics (Pitt et al., 1995; Göbel et al., 2007; Hwang et al., 2016; Müller et al., 2020; Spahr et al., 2020; Werbowski et al., 2021; Grebel et al., 2013). As a result, several national and international databases have compiled a list of recurrent stormwater contaminants. A prominent example is the National Stormwater Quality Database (NSQD), which contains stormwater quality data from over 9000 storm events and 200 municipalities across the U.S. (Pitt et al., 2018). Regarding stormwater regulations, the EU Water Framework Directive (WFD; Directive 2000/60/EC), along with its recent amendments, strives to increase the list of contaminants in stormwater monitoring programs to protect aquatic ecosystems. In this regard, stormwater discharges in the U.S. must be in compliance with the Federal National Pollutant Discharge Elimination System (NPDES) program implemented by the United States Environmental Protection Agency (US EPA, 2010).
Although stormwater contamination is considered as a nonpoint source (i.e., diffuse contamination), several attempts to classify stormwater pollution sources have been reported in the literature. In short, stormwater contamination depends on a combination of topographic factors, climatic conditions, and anthropogenic activities. Most studies of contaminant quantification in urban runoff have identified the following contributors: (i) atmospheric deposition (Huston et al., 2009; Deng, 2021); (ii) land cover and land use (Burant et al., 2018; Wicke et al., 2021), which consists of surface-related (build-up and wash-off contamination from the built environment and green spaces) along with economic activity-related activities (issuing from processing, manufacturing, and construction activities) (Hobbie et al., 2017; Brown and Peake, 2006); (iii) transport-related activities (e.g., tire and brake erosions, oil losses, vehicle body paint, exhaust emissions, pavement erosion, road maintenance) (Lundy et al., 2012); and (iv) waste-related activities (e.g., licit/illicit littering) (Chevalier et al., 2018).
To the best of our knowledge, there is only one germinal study characterizing stormwater quality from Barcelona (Llopart-Mascaró et al., 2010). Unfortunately, the limited data available (i.e., collection at few specific locations), together with the appearance of new materials and sources of pollutants, request a re-assessment of the urban contaminants in the runoff. Therefore, many data gaps remain regarding the presence and sources of contaminants, along with possible mitigation strategies to reduce receiving water impairment. In this context, its built blue-green infrastructure (BGI), also called sustainable urban drainage systems (SuDS) or best management practices (BMP), have never been assessed for their stormwater contaminant treatment capacity.
Although our ongoing research includes nutrients, trace metals and organic contaminants of emerging concern (e.g., urban-related trace organics), this preliminary work mainly investigates the occurrence of trace metals in rainwater, runoff and BGI outlets. It is important to note that stormwater can generate aquatic toxicity due to heavy metals (Karlsson et al., 2010). The specific objectives of this article were: (i) to investigate the stormwater quality collected at different locations and assess the effect of the immediate catchment area performing a multivariate analysis; and (ii) to conduct an initial evaluation of the capacity of blue-green infrastructure towards trace metals detected in the adjacent areas. Stormwater runoff quality data can define its potential uses and limitations, be used to build large-scale hydrogeologic models to predict contaminant occurrence (specially because a significant spatiotemporal lack of data exists). Such models could be used to guide future sampling efforts, to update our historical data on stormwater quality (if available), to identify areas of high risk for human exposure, and to take corrective measures. Therefore, the results of this study, once completed, will improve contaminant modelling within the water cycle, identification of pollutant sources, and deployment of specific control measures.
2.1 Study site
The area of study includes the city of Barcelona, which is located at the north-eastern part of the Iberian Peninsula (coordinates; 41∘23′19.64′′ N, 2∘9′32.36′′ E). This area covers: the Collserola range (west), and the Mediterranean Sea (east and southeast) (Fig. 1). It is limited by two rivers: the Llobregat and the Besòs at the south-west and north-east, respectively. Most of the area of study has been significantly modified by anthropogenic activity, and many exposures of the geology have been covered or destroyed. Four zones can be distinguished regarding the study area hydrogeological characteristics: (i) the Collserola Mountains (mainly composed by metamorphic materials); (ii) the Llobregat river delta (alluvial fans); (iii) Besòs river delta (alluvial fans); and (iv) the Barcelona plain (alluvial fans) (Tubau et al., 2017). Barcelona climate is typically Mediterranean, i.e., “Csa” according to the Köppen–Geiger climate classification. It has a temperate climate, characterized by mild winters and dry summers. Most of the precipitation occurs in spring and fall. The average precipitation is approximately 600 mm yr−1 but presents high variability within the year (Barro et al., 2002). Flash floods and heavy rainfalls can be common in the study area (Llasat, 2004). Barcelona rainfall patterns, an old combined sewer system (single pipeline collecting stormwater runoff and domestic sewage), terrain slope, along with a high imperviousness degree (approximately 70 % of the municipal area), cause severe floods (i.e., personal injuries and damage to property) and combined sewer overflows (Martínez-Gomariz et al., 2021). Finally, Barcelona has very intense vehicle traffic (Querol et al., 2004). Its estimated average daily traffic (ADT) was obtained from the Barcelona City Council.
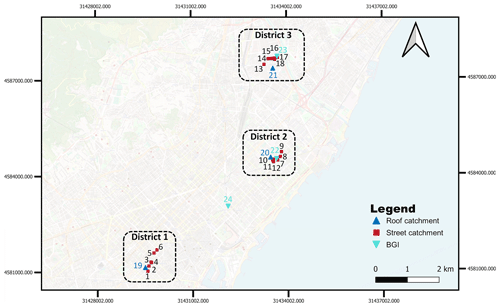
Figure 1Location of the sampling sites located in three different Barcelona districts (Districts 1, 2 and 3 stand for “Eixample”, “St. Martí” and “St. Andreu”, respectively). Seven sampling campaigns were conducted during Spring 2022 in three different catchment areas: roof (dark blue triangles), street (red squares), and blue-green infrastructure effluent (light blue inverted triangles). The World Geodetic System (WGS84) was used as reference coordinate system. Source for basemap: © ArcGIS (ESRI).
2.2 Sampling and analytical methods
Nearly 200 “first-flush” stormwater samples were manually collected using a 1 L self-lock system in 7 field campaigns during the period ranging from March to April 2022. A reported extensive analysis of the Barcelona precipitation series identified this period as the second rainy season (Rodriguez et al., 1999). Up to 24 sites scattered in three different districts of Barcelona (Eixample, Sant Marti, and Sant Andreu) were chosen (Fig. 1). Sampling sites included: 3 roofs, 13 conventional streets with vehicle traffic (i.e., traffic roads), 5 pedestrian streets (vehicle-free zones), and 3 drainpipes (effluent) from BGI located in the vicinity of the sampled streets. As our sampling program only included discrete samples, removal efficiency by BGI could not be estimated. Electrical conductivity (EC µS cm−1), pH, and dissolved oxygen (DO; mg L−1) were in-situ measured using a Hanna Instruments™HI98494 portable multiparameter. The main analyses were performed at IDAEA-CSIC facilities. Prior to off-site analysis, samples for trace metals were filtered with 0.45 µm NYL syringe filter, acidified to 1 % HNO3, stored at 4 ∘C until day of the analysis (< 3 d). Dissolved trace metal concentrations (Cr, Ni, Cu, Zn, Cd, and Pb) were determined by inductively coupled plasma mass spectrometry (ICP-MS). For the dissolved organic carbon (DOC) quantification, samples were filtered through a 0.45 µm GF filter, acidified to pH below 2 units with HCl, and kept refrigerated until analysis (< 2 d). DOC was measured with a Shimadzu TOC-VCSH.
2.3 Statistical analysis and data treatment
Multivariate Principal Component Analysis (PCA) was performed over the data matrix, consisting of the water quality parameters and the trace metal concentrations at each sampling site. PCA is a multivariate data analysis tool that aims to explain the variability of data by converting a suite of potentially correlated measured variables into a reduced set of uncorrelated variables, known as principal components (PCs) (Farnham et al., 2002). Multivariate analysis was carried over by using the PLS 9.1 Toolbox (Eigenvector Research Ltd. Manson, WA, USA) under the MATLABR2010b (MathWorks, Natick, MA, USA). Before the multivariate analysis was carried out, data pretreatment included an autoscaling step, and values below the limit of detection (LOD) were removed (e.g., Cd). Two-way analysis of variance (ANOVA) was conducted to evaluate differences between multiple groups (α=0.05) using GraphPad Prism® version 9 2020.
3.1 Stormwater runoff quality and relationships between contaminant and catchment area
Overall, we detected a wide range of trace metals in the nearly 200 stormwater samples during the 7 storm events investigated. Table 1 shows the trace metal concentrations (mean, standard deviation, maximum and minimum values) together with other water quality parameters in stormwater samples collected at four different locations: roofs, conventional streets (i.e., streets with vehicular traffic), pedestrian streets (vehicle-free zones), and blue-green infrastructures drainpipes (i.e., effluent). Measured metal concentrations were within the few to hundred microgram-per-liter levels reported in the NSQD (Pitt et al., 2018). Zn, which is primarily related to car tire abrasion, showed the highest concentrations (mean: 104 µg L−1), while Cd (related to car exhaust, brakes, and tires) was below the quantification limit (0.01 µg L−1) and was excluded in our analysis. Pb concentration (associated to car body paints) was also lower than reported by the NSQD. In fact, only Cu and Zn exceed USEPA aquatic life benchmarks (Grebel et al., 2013). Similarly, Davis et al. (2001) reported a similar sequence of metal concentrations in urban and highway runoff: Zn > Cu > Cd (with the exemption of Pb).
Table 1Average concentration of selected trace metals (µg L−1; parts-per-billion) and complementary water quality parameters of Barcelona stormwater runoff. Standard deviations are given after ±. Maximum and minimum values are given between brackets.
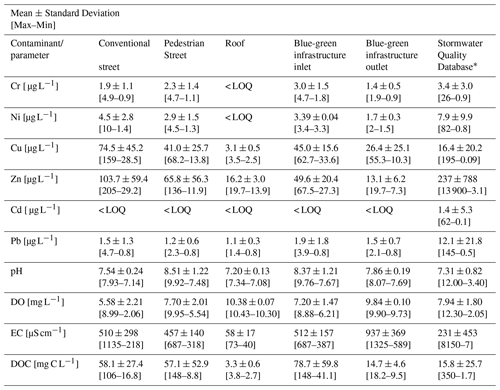
∗ Trace metal concentrations reported by the National Stormwater Quality Database (Pitt et al., 2018). This database contains stormwater quality data from over 9000 storm events and 200 municipalities across the U.S. (comprising different land uses).
Prior to multivariate studies, we conducted a calculation of the pair-wise correlations among the different variables. Although it is only an exploratory tool, it revealed some relationships between the 11 variables studied. Most studied trace metals (e.g., Cr, Ni, Cu and Zn) showed negative correlations with dissolved oxygen (Pearson correlation r values ranging from −0.4 to −0.95), and positive correlations with ADT (r values ranging from 0.5 to 0.6), electrical conductivity (r values ranging from 0.3 to 0.6), DOC (r values ranging from 0.5 to 0.6) and UVA254 (specific UV absorbance at 254 nm, r values ranging from 0.3 to 0.7). Anova tests also showed significant differences (p<0.05) among the type of catchment area (roof, conventional, pedestrian, and blue-green infrastructure effluent).
Results from the multivariate analysis indicated that 6 principal components (PCs) captured almost the entire variance of the data matrix (> 95 %). Eventually, an initial 2-PC model was chosen because the first two PCs captured about 70 % of total variance. This allows a preliminary assessment of the dependences among variables and provides a simplified interpretation of the observed relationships. Prior to building the model, the 24 sampling sites were initially classified in 4 classes: roofs, conventional streets (automobile-oriented streets), pedestrian streets, and outlets of blue-green infrastructures. Figure 2 depicts the biplot of the loadings and scores in the PC space, which allows the identification of the variables responsible for the observed differences in the sample scores.
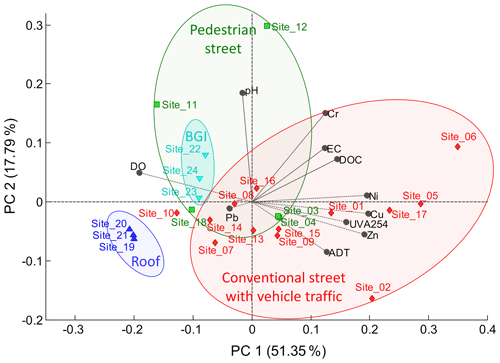
Figure 2Biplot of the loadings and scores in the principal component (PC) space. The dominant (PC1) and secondary (PC2) modes are displayed on the x- and y-axis, respectively. Samples are shown in different classes: conventional street with vehicular traffic (red diamonds), pedestrian street (green squares), roof (dark blue triangles) and blue-green infrastructure effluent (light blue inverted triangle). Classes have been clustered using ellipses with for clarity.
In our PCA model, the four different classes of samples were clustered in the scores plot (we connected them using ellipses for reader's clarity in Fig. 2), clearly highlighting the differences among these groups. Figure 2 shows that roof, BGI, and most vehicle-impacted conventional street samples could be easily grouped in different clusters, while pedestrian sites had clear differences among them. For instance, sites 3 and 4 fell well within the cluster of conventional streets, thought they are classified by the City Council as pedestrian streets (estimated ADT < 100 vehicles day−1). The biplot also demonstrates that sites 3 and 4 fell next to sites 1, 9 and 15 with estimated daily traffic loads ranging from 1000–7000 vehicles day−1. Although vehicle traffic has been restricted in sites 3 and 4 since 2017, these counterintuitive results are likely due to high unauthorized vehicle traffic (mostly non-local drivers and truck deliveries) in this central part of the city (District 1, Fig. 1). As expected, other vehicle-free streets far away from downtown (i.e., Districts 2 and 3; sites 11, 12, and 18) were located closer to the roof and the BGI clusters which were the least contaminated sites (Fig. 2). According to local regulation, these pedestrian streets are restricted areas which only allow one-lane traffic from local resident vehicles, bikes and emergency vehicles circulating at a maximum speed of 10–20 km h−1, while in two-lane unrestricted conventional streets the maximum speed is set at 30–50 km h−1 (Rueda, 2019). Interestingly, sample 10 showed very low contamination although the street is located at a semi-abandoned industrial area but with traffic volume (i.e., ADT) similar than pedestrian streets (e.g., sites 11, 12, and 18). This confirms previous studies that emphasized that specific characteristics of the studied surface has more impact on water quality than traditional land use classifications (e.g., industrial, commercial, and residential) (Liu et al., 2013; Burant et al., 2018).
The dominant PC (PC1; 51 % explained variance) displayed positive loadings for most trace metal contaminants (with the notable exemption of Pb), vehicle traffic volume (ADT), organic matter content (DOC and UVA254), and EC. PC1 also shows that DO showed relatively high negative loadings. In addition, PC2, capturing 18 % of the explained variance, was mainly positively loaded by pH, together with EC and DOC. PC1 and PC2 correlated the trace metal concentrations (i.e., pollution) with other variables (e.g., ADT, DOC, EC, DO). As aforementioned, extant literature on vehicle-related contamination has associated Cr, Ni, Cu, Zn, Cd and Pb with emissions from automobile exhausts, wear (e.g., body, engine, and tires), wash-off processes, and fluid leaks (e.g., engine oil) (Müller et al., 2020). Therefore, metals are indicative of areas congested with vehicles (Ferreira et al., 2016; Nawrot et al., 2020). Several previous works emphasized that the grade of congestion (generally quantified by ADT estimations) significantly influenced the metal concentrations, but other site factors should also be taken into account (road design, traffic lights, signs, etc.) (Horstmeyer et al., 2016; Opher and Friedler, 2010). Pb has been linked to vehicle emissions and extensively monitored in urban environments due to its high toxicity (Goyer, 1993). However, our results show that lead, located close to the biplot origin, had a low contribution to the overall variation of the data. In fact, Pb was not correlated to the other studied metal contaminants, probably because it has been banned in motor vehicle gasoline since 2000 (Kayhanian et al., 2007).
Stormwater runoff organic fraction (i.e., DOC) along with DO concentration seem to also explain the observed site differences. This is particularly true for the vehicle-impacted runoff samples collected at sites 5, 6 and 17 which were heavily polluted with metals, contained high DOC, and were anoxic. This low oxygen (i.e., microbial activity) concomitant with high organic matter presence can be attributed to sewage sewer overflows (SSO) that could have contaminated stormwater runoff (Gasperi et al., 2012). As aforementioned, Figure 2 also unveils a correlation between DOC and metal concentrations. Several authors have reported strong binding (i.e., complexation reactions) between heavy metals and dissolved organic matter (Zhu et al., 2022; Wei et al., 2020). Based on our results, DOC (UVA254) and EC could be used as early indicator for traffic-impacted stormwater (Yu et al., 2018). In contrast, samples taken at roofs, pedestrian streets, and blue-green infrastructure outlets seem negatively correlated with pollution (low metal content, DOC, UVA254, EC, together with high DO concentrations) as indicated by their relative positions in the biplot (Fig. 2).
Bioretention cells, clustered in the PC space (sites 22 to 24; Fig. 2), showed that samples were less contaminated by metals than the samples taken at the inlet of the blue-green infrastructure (Table 1). BGI mean effluent metal concentrations ranged between 1.4 and 26.4 µg L−1. This corroborates the significant differences (p<0.05) observed in our preliminary ANOVA analyses especially for DO, DOC, Ni, Cu, and Zn. Overall, these results are in agreement with similar field studies that studied metal concentrations at the BGI inlets and outlets (Trowsdale and Simcock, 2011). For instance, the 2020 Urban BMP database performance summary reported that bioretention systems and grass bioswales can exhibit a metal removal efficiencies ranging from 40 % to 80 % (Clary et al., 2020). Although Cu concentrations in this work were lower at the BGI effluent samples compared to inlet ones, our results also highlighted that Cu had the highest concentration level among studied metals, with effluent concentrations ranging from 10 to 55 µg L−1. This high Cu effluent concentrations has been reported in the BMP Database and similar studies which emphasized that BGI could become a Cu source eventually (i.e., negative removal) (Trowsdale and Simcock, 2011). Although Cu exports are concerning due to its well-known environmental toxicity (Rehman et al., 2019), studies have shown that dissolved Cu leaching from compost-amended bioretention systems is mainly complexed to DOM, resulting in a reduced bioavailability for aquatic organisms (Chahal et al., 2016).
A preliminary assessment of the relationships between urban stormwater pollution and a set of sites located at three different districts has been conducted. Our initial multivariate study highlighted the differences between catchment areas (roofs, streets with vehicle or pedestrian traffic, and BGI outlets). Conventional streets with higher traffic volumes (i.e., ADT) resulted in stormwater runoff with higher trace metal concentrations and other water quality parameters, such as, DOC, UVA254, EC, but lower DO concentrations. In addition, this study also shows that blue-green infrastructure (also called sustainable urban drainage systems, SuDS) can provide effective significant means of trace metal concentration reduction, improving stormwater quality. In conclusion, our results emphasize the relevance of catchment area in the quality of rain or stormwater, and it emphasizes the benefits of using blue-green infrastructure as pollution-control tools.
The data for this work will be available in the online Mendeley repository under a Standard Creative Commons Attribution License (CC BY) 4.0 International. Data will be available free of charge and will also be provided upon request by the corresponding author.
MT, EV, and LS secured the funding; MT, EVS, MJC, and LS designed the study; MJC granted access to sampling points; MT, DS, and JX executed the field campaigns and performed the chemical analyses; MT performed data analysis and drafted the manuscript; MT, LS, and EVS reviewed and edited the manuscript.
The contact author has declared that none of the authors has any competing interests.
Publisher’s note: Copernicus Publications remains neutral with regard to jurisdictional claims in published maps and institutional affiliations.
This article is part of the special issue “Quality and quantity issues in urban hydrogeology (EGU2022 HS8.2.8 session)”. It is a result of the EGU General Assembly 2022, Vienna, Austria, 23–27 May 2022.
Laura Scheiber would like to thank the Ibero-American Programme of Science and technology for development (CYTED – Programa de ciencia y tecnologia para el desarrollo) under project 719RT0585. Moreover, Laura Scheiber thank the support from the Barcelona city council through the Award for Scientific Research into Urban Challenges in the City of Barcelona 2020 (reference code: 21S04651).
This research has been supported by the Agència de Gestió d'Ajuts Universitaris i de Recerca (Beatriu de Pinós 2020 (grant no. 2020BP00280)). This work was supported by the Barcelona City Council through the
“Scientific Research into Urban Challenges in the City of Barcelona 2020
Award” (grant no. 21S04652).
We acknowledge support of the publication fee by the CSIC Open Access Publication Support Initiative through its Unit of Information Resources for Research (URICI).
This paper was edited by Miao Jing and reviewed by RamyaPriya Ramesh and one anonymous referee.
Barcelona City Council: Technical guide to design SUDS in Barcelona, GUIA TÈCNICA PER AL DISSENY DE SISTEMES DE DRENATGE URBÀ SOSTENIBLE (SUDS), https://ajuntament.barcelona.cat/ecologiaurbana/sites/default/files/PlecPrescripcionsTecniquesDrenatge_Guia.pdf (last access: Feb 2023), 2020.
Barro, J. R., Ortega, L., Salamero, M., and Escaler, I.: The Spanish National CSO Measurement Project (Promedsu) as a Base for Real Time Sewer Management Depending on Water Quality – Barcelona's Case, in: Global Solutions for Urban Drainage, 1–12, https://doi.org/10.1061/40644(2002)326, 2002.
Björklund, K., Bondelind, M., Karlsson, A., Karlsson, D., and Sokolova, E.: Hydrodynamic modelling of the influence of stormwater and combined sewer overflows on receiving water quality: Benzo (a) pyrene and copper risks to recreational water, J. Environ. Manag., 207, 32–42, 2018.
Brown, J. N. and Peake, B. M.: Sources of heavy metals and polycyclic aromatic hydrocarbons in urban stormwater runoff, Sci. Total Environ., 359, 145–155, https://doi.org/10.1016/j.scitotenv.2005.05.016, 2006.
Burant, A., Selbig, W., Furlong, E. T., and Higgins, C. P.: Trace organic contaminants in urban runoff: Associations with urban land-use, Environ. Pollut., 242, 2068–2077, https://doi.org/10.1016/j.envpol.2018.06.066, 2018.
Chahal, M. K., Shi, Z., and Flury, M.: Nutrient leaching and copper speciation in compost-amended bioretention systems, Sci. Total Environ., 556, 302–309, 2016.
Chevalier, Q., El Hadri, H., Petitjean, P., Bouhnik-Le Coz, M., Reynaud, S., Grassl, B., and Gigault, J.: Nano-litter from cigarette butts: Environmental implications and urgent consideration, Chemosphere, 194, 125–130, 2018.
Clary, J., Jones, J., Leisenring, M., Hobson, P., and Strecker, E.: International Stormwater BMP Database: 2020 Summary Statistics, https://bmpdatabase.org/ (last access: February 2023), 2020.
Davis, A. P., Shokouhian, M., and Ni, S.: Loading estimates of lead, copper, cadmium, and zinc in urban runoff from specific sources, Chemosphere, 44, 997–1009, 2001.
Deng, Y.: Pollution in rainwater harvesting: A challenge for sustainability and resilience of urban agriculture, Journal of Hazardous Materials Letters, 2, 100037, https://doi.org/10.1016/j.hazl.2021.100037, 2021.
Farnham, I. M., Singh, A. K., Stetzenbach, K. J., and Johannesson, K. H.: Treatment of nondetects in multivariate analysis of groundwater geochemistry data, Chemometr. Intell. Lab., 60, 265–281, 2002.
Ferreira, A. J., Soares, D., Serrano, L. M., Walsh, R. P., Dias-Ferreira, C., and Ferreira, C. S.: Roads as sources of heavy metals in urban areas. The Covões catchment experiment, Coimbra, Portugal, J. Soils Sediments, 16, 2622–2639, 2016.
Gasperi, J., Zgheib, S., Cladière, M., Rocher, V., Moilleron, R., and Chebbo, G.: Priority pollutants in urban stormwater: Part 2 – Case of combined sewers, Water Res., 46, 6693–6703, 2012.
Göbel, P., Dierkes, C., and Coldewey, W. G.: Storm water runoff concentration matrix for urban areas, J. Contam. Hydrol., 91, 26–42, 2007.
Goyer, R. A.: Lead toxicity: current concerns, Environ. Health Perspect., 100, 177–187, 1993.
Grebel, J. E., Mohanty, S. K., Torkelson, A. A., Boehm, A. B., Higgins, C. P., Maxwell, R. M., Nelson, K. L., and Sedlak, D. L.: Engineered Infiltration Systems for Urban Stormwater Reclamation, Environ. Eng. Sci., 30, 437–454, https://doi.org/10.1089/ees.2012.0312, 2013.
Hobbie, S. E., Finlay, J. C., Janke, B. D., Nidzgorski, D. A., Millet, D. B., and Baker, L. A.: Contrasting nitrogen and phosphorus budgets in urban watersheds and implications for managing urban water pollution, P. Natl. Acad. Sci. USA, 114, 4177–4182, 2017.
Horstmeyer, N., Huber, M., Drewes, J. E., and Helmreich, B.: Evaluation of site-specific factors influencing heavy metal contents in the topsoil of vegetated infiltration swales, Sci. Total Environ., 560–561, 19–28, https://doi.org/10.1016/j.scitotenv.2016.04.051, 2016.
Huston, R., Chan, Y. C., Gardner, T., Shaw, G., and Chapman, H.: Characterisation of atmospheric deposition as a source of contaminants in urban rainwater tanks, Water Res., 43, 1630–1640, 2009.
Hwang, H.-M., Fiala, M. J., Park, D., and Wade, T. L.: Review of pollutants in urban road dust and stormwater runoff: part 1. Heavy metals released from vehicles, International Journal of Urban Sciences, 20, 334–360, https://doi.org/10.1080/12265934.2016.1193041, 2016.
Karlsson, K., Viklander, M., Scholes, L., and Revitt, M.: Heavy metal concentrations and toxicity in water and sediment from stormwater ponds and sedimentation tanks, J. Hazard. Mater., 178, 612–618, 2010.
Kayhanian, M., Suverkropp, C., Ruby, A., and Tsay, K.: Characterization and prediction of highway runoff constituent event mean concentration, J. Environ. Manage., 85, 279–295, 2007.
Liu, A., Egodawatta, P., Guan, Y., and Goonetilleke, A.: Influence of rainfall and catchment characteristics on urban stormwater quality, Sci. Total Environ., 444, 255–262, 2013.
Liu, J., Yang, H., Gosling, S. N., Kummu, M., Flörke, M., Pfister, S., Hanasaki, N., Wada, Y., Zhang, X., and Zheng, C.: Water scarcity assessments in the past, present, and future, Earths Future, 5, 545–559, 2017.
Llasat, M. D. C.: The vulnerability in Catalonia and the social perception, La Houille Blanche, 90, 71–75, https://doi.org/10.1051/lhb:200406009, 2004.
Llopart-Mascaró, A., Ruiz, R., Martínez, M., Malgrat, P., Rusiñol, M., Gil, A., Suárez, J., Puertas, J., del Rio, H., and Paraira, M.: Analysis of rainwater quality. Towards a sustainable rainwater management in urban environments-Sostaqua Project, in: 7ème Conférence internationale sur les techniques et stratégies durables pour la gestion des eaux urbaines par temps de pluie/7th International Conference on sustainable techniques and strategies for urban water management, 1–10, hal-03296542, 2010.
Lundy, L., Ellis, J. B., and Revitt, D. M.: Risk prioritisation of stormwater pollutant sources, Water Res., 46, 6589–6600, 2012.
Luthy, R. G. and Sedlak, D. L.: Urban Water-Supply Reinvention, Daedalus, 144, 72–82, https://doi.org/10.1162/DAED_a_00343, 2015.
Martínez-Gomariz, E., Forero-Ortiz, E., Russo, B., Locatelli, L., Guerrero-Hidalga, M., Yubero, D., and Castan, S.: A novel expert opinion-based approach to compute estimations of flood damage to property in dense urban environments. Barcelona case study, J. Hydrol., 598, 126244, https://doi.org/10.1016/j.jhydrol.2021.126244, 2021.
Müller, A., Österlund, H., Marsalek, J., and Viklander, M.: The pollution conveyed by urban runoff: a review of sources, Sci. Total Environ., 709, 136125, https://doi.org/10.1016/j.scitotenv.2019.136125, 2020.
Nawrot, N., Wojciechowska, E., Rezania, S., Walkusz-Miotk, J., and Pazdro, K.: The effects of urban vehicle traffic on heavy metal contamination in road sweeping waste and bottom sediments of retention tanks, Sci. Total Environ., 749, 141511, https://doi.org/10.1016/j.scitotenv.2020.141511, 2020.
Opher, T. and Friedler, E.: Factors affecting highway runoff quality, Urban Water J., 7, 155–172, https://doi.org/10.1080/15730621003782339, 2010.
Pitt, R., Field, R., Lalor, M., and Brown, M.: Urban stormwater toxic pollutants: assessment, sources, and treatability, Water Environ. Res., 67, 260–275, 1995.
Pitt, R., Maestre, A., and Clary, J.: The National Stormwater Quality Database (NSQD), Version 4.02, Department of Civil and Environmental Engineering, 2018.
Querol, X., Alastuey, A., Ruiz, C. R., Artiñano, B., Hansson, H. C., Harrison, R. M., Buringh, E., ten Brink, H. M., Lutz, M., Bruckmann, P., Straehl, P., and Schneider, J.: Speciation and origin of PM10 and PM2.5 in selected European cities, Atmos. Environ., 38, 6547–6555, https://doi.org/10.1016/j.atmosenv.2004.08.037, 2004.
Rehman, M., Liu, L., Wang, Q., Saleem, M. H., Bashir, S., Ullah, S., and Peng, D.: Copper environmental toxicology, recent advances, and future outlook: a review., Environ. Sci. Pollut. Res. Int., 26, 18003–18016, https://doi.org/10.1007/s11356-019-05073-6, 2019.
Rodriguez, R., Llasat, M.-C., and Wheeler, D.: Analysis of the Barcelona precipitation series 1850–1991, Int. J. Climatol., 19, 787–801, 1999.
Rueda, S.: Superblocks for the design of new cities and renovation of existing ones: Barcelona's case, Integrating Human Health into Urban and Transport Planning: A Framework, 135–153, https://doi.org/10.1007/978-3-319-74983-9_8, ISBN 978-3-319-74982-2, 2019.
Spahr, S., Teixidó, M., Sedlak, D. L., and Luthy, R. G.: Hydrophilic trace organic contaminants in urban stormwater: occurrence, toxicological relevance, and the need to enhance green stormwater infrastructure, Environ. Sci.-Water Res. Technol., 6, 15–44, https://doi.org/10.1039/C9EW00674E, 2020.
Trowsdale, S. A. and Simcock, R.: Urban stormwater treatment using bioretention, J. Hydrol., 397, 167–174, 2011.
Tubau, I., Vázquez-Suñé, E., Carrera, J., Valhondo, C., and Criollo, R.: Quantification of groundwater recharge in urban environments, Sci. Total Environ., 592, 391–402, https://doi.org/10.1016/j.scitotenv.2017.03.118, 2017.
US EPA: United States Environmental Protection Agency (USEPA): Stormwater Discharges from Municipal Sources, https://www.epa.gov/npdes/stormwater-discharges-municipal-sources-resources (last access: February 2023), 2010.
Wei, J., Tu, C., Yuan, G., Zhou, Y., Wang, H., and Lu, J.: Limited Cu(II) binding to biochar DOM: Evidence from C K-edge NEXAFS and EEM-PARAFAC combined with two-dimensional correlation analysis, Sci. Total Environ., 701, 134919, https://doi.org/10.1016/j.scitotenv.2019.134919, 2020.
Werbowski, L. M., Gilbreath, A. N., Munno, K., Zhu, X., Grbic, J., Wu, T., Sutton, R., Sedlak, M. D., Deshpande, A. D., and Rochman, C. M.: Urban stormwater runoff: a major pathway for anthropogenic particles, black rubbery fragments, and other types of microplastics to urban receiving waters, ACS ES&T Water, 1, 1420–1428, 2021.
Wicke, D., Matzinger, A., Sonnenberg, H., Caradot, N., Schubert, R.-L., Dick, R., Heinzmann, B., Dünnbier, U., von Seggern, D., and Rouault, P.: Micropollutants in urban stormwater runoff of different land uses, Water, 13, 1312, https://doi.org/10.3390/w13091312, 2021.
Yu, Q., Yin, H., Wang, K., Dong, H., and Hou, D.: Adaptive Detection Method for Organic Contamination Events in Water Distribution Systems Using the UV-Vis Spectrum Based on Semi-Supervised Learning, Water, 10, 1566, https://doi.org/10.3390/w10111566, 2018.
Zhu, P., Knoop, O., and Helmreich, B.: Interaction of heavy metals and biocide/herbicide from stormwater runoff of buildings with dissolved organic matter, Sci. Total Environ., 814, 152599, https://doi.org/10.1016/j.scitotenv.2021.152599, 2022.