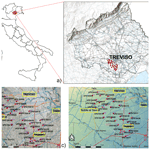
Characterization of groundwater recharge through tritium measurements
Antonietta Rizzo
Stefano Salvi
Alessandro Pozzobon
Elena Marrocchino
Carmela Vaccaro
After the nuclear weapons testing in the 1950s and 1960s, the atmospheric tritium levels have almost returned to natural levels (pre-1950 levels), which means that tritium is becoming more effective in determining transit times in hydrological systems. It has also been demonstrated that tritium is a non-reactive noble gas and in water is not subject to chemical reactions, absorption, or dissolution/precipitation processes, so it is conservative of the geochemical fingerprint of the source. In addition, it is used as an effective tracer of water contamination by landfill leachate, allowing to detect mixing percentages of the leachate up to levels not achievable with normal chemical analyses (less than 1 %). The purpose of the present work is to deepen the knowledge of the hydrogeochemical characteristics of the aquifers in the pedemontana area of the Venetian plain, and to formulate a conceptual framework of underground water circulation, aimed at understanding phenomena of contamination by toxic metals, harmful to human health. Tritium concentration analyses were performed on selected samples of groundwater collected in different wells in the south part of Treviso city to correlate the young age of the water recharge. The abundance of young waters, in fact, indicates a vulnerability of the aquifer to climate change with respect to possible contributions of surface waters, in particular to prolonged drought periods which could induce salinization dynamics.
- Article
(4832 KB) - Full-text XML
- BibTeX
- EndNote
Tritium (3H) is a beta-emitting radioactive isotope of hydrogen which decays into Helium-3 (). The half-life of the unstable tritium nucleus is of 4500 d (12.3 years), which is very short on the radioactive timescale (MacMahon, 2006).
Tritium concentration in water is expressed as tritium units (TU), that correspond to a tritium atom per 1018 hydrogen atoms, that is 0.119 Bq L−1 of water. The content of natural tritium in water vapour in the stratosphere was estimated by Ehhalt et al. (2002) and by Fourré et al. (2006) in TU, a value of several orders of magnitude higher than the level of natural tritium present in precipitation (a few TU). Cauquoin et al. (2015) have argued that this difference is the result of a higher production rate in the stratosphere and a lower water content in it.
The only source of natural tritium in the hydrosphere is due to the meteoric supply, which can vary worldwide from 2 to 25 TU and, in the Central Mediterranean area, is around an average annual values of 5–8 TU.
Cauquoin et al. (2015) have formulated a model of the distribution of the average annual concentration of tritium in precipitations, thank to that it emerged that the precipitations in the sampling area have on average concentrations of tritium with maximum values of 5–6 TU, which can increase up to 7 for contributions from mountain areas.
The tritium atoms produced in the atmosphere, reacting with atmospheric oxygen form tritiated water in the forms of HTO, DTO and T2O which, having a greater molecular mass than the H2O molecule, has a shorter residence time in the atmosphere and tends to be removed with precipitation (Rozanski et al., 1991). The short period of residence in the atmosphere and the short period of decay leads to low and nearly constant tritium concentration in rain, as there is a balance between the rate of formation, the removal by the rains and the total quantity of natural tritium in the environment which is Ci (DOE Handobook, 2008).
Considering that high Tritium level arising from the nuclear weapons testing period were detected, the underground waters with a long residence time in the subsoil and infiltrated before 1950 are below the threshold of analytical detectability for tritium. The concentrations of tritium in the atmosphere have been altered by the war events and nuclear experiments that have introduced, from 1952 to 1963 (UNSCEAR, 2000), anthropogenic tritium into the atmosphere (UNSCEAR, 2016). These events modified the geochemical footprint of meteoric precipitations, with meaningful increases that have been interrupted since 1963, when an international treaty (PTBT) banned all nuclear tests in the atmosphere. The end of nuclear experimentation has produced a gradual decrease in the concentration of tritium in the atmosphere and during the last 25 years' the equilibrium conditions of tritium have returned. Today, the tritium measurements show very low-level concentration which can be used to date waters with residence times of up to 100–150 years (Morgenstern et al., 2010, 2015).
Observing the data recorded in the Locarno station of the GNIP network, it is also possible to observe a seasonal variability of the content of tritium in rainwater, which records, on average in the period 2000–2009, an increase from January to May and a decrease from June to December (IAEA/WMO, 2019). The values, that are around 4 TU in January, can reach a peak of 9.5 TU in concomitance with precipitation coming from Arctic areas. The growing influence of cosmogenic production at the poles is due as a consequence of the injection of stratospheric air masses with higher tritium content and with very low water vapour content.
Underground and in the absence of recharge of meteoric waters, a continuous loss of tritium due to its decay occurs.
In case of recharging of underground reserves by meteoric water rich in tritium, the concentration reflects the balance between the loss due to decay and the contribution of meteoric water enriched with tritium (Bryan et al., 2016; Cartwright et al., 2017).
Furthermore, it has been shown that tritium follows the water flow without being subjected to chemical reactions, absorption or dissolution/precipitation processes, so it is conservative of the geochemical footprint of the source. For these reasons, analysing the concentrations of tritium in the aquifer water can give important information regarding the knowing on the recharge times of the aquifer: the more the concentrations of tritium are similar or equal to atmospheric ones, the more recent the recharge of the aquifer is; vice versa, if the tritium concentrations in the aquifer are lower than atmospheric ones, it means that the aquifer is not subject to superficial meteoric infiltration or that the aquifer is very slowly recharging. This information is very important to understand, for example, if the aquifer is overexploited or not by the local population.
In addition, it is used as an effective tracer of water contamination by landfill leachate, allowing to detect percolate mixing percentages up to levels not achievable with normal chemical analyses (lower 1 %).
Although the Treviso plain has been extensively investigated, its hydro-structure is not perfectly defined and, consequently, the relations between the qualitative characteristics of the multi-aquifer system and the variations in petrophysical properties are not clear. This knowledge is important to better quantify the impact of the rainfall regime due to climate change and its ability to ensure not only the balance between recharging and exploitation of water bodies, but also maintaining the qualitative characteristics of the resource.
Several studies have contributed to fill the knowledge gap concerning the dynamics of aquifer feeding (Baruffi et al., 2012; Gattacceca et al., 2009) and the possible interactions at regional and local scale between low salinity freshwater and brackish waters. The latter ones may derive from intrusion of seawater into the continental environment caused to over-exploitation dynamics of the aquifer.
In this view, the use of tritium analyses could be useful to date (Guo et al., 2019; Silva and Cota, 2020) and characterize different aquifers (Morgenstern et al., 2010, 2015; Jerbi et al., 2019).
In addition, stable isotopes could be a supplementary tool used to characterize water sources (Ala-aho et al., 2018; Glibert et al., 2019). 2H and 18O are widely used in hydrological studies (Gibson et al., 2020; Yang et al., 2020) to trace fluxes of water in different hydrological conditions (Chen et al., 2020; Zhou et al., 2021) or to characterize the different contribution of streamflow components (Kim et al., 2017; Tao et al., 2021), despite the evaporation processes may change these processes (Schmieder et al., 2018; Boral et al., 2019). Improvement of technological techniques, as laser spectroscopy, helped increase the information of these tracers in different water compartments (Evaristo et al., 2017; Mello et al., 2020). Within this context, we tested the reliability of water stable isotopes, in addition to hydrochemical parameters, in order to identify (i) water origin, being the isotopic signature of groundwater inherited especially from rainfall; (ii) possible identification of anthropogenic pollutions in relation with tritium value (Abdullah et al., 2018; Bronić and Barešić, 2021).
For this purpose, we investigated tritium and the ionic and isotopic composition (18O and 2H) of the water samples collected in different kind of wells in the south part of Treviso plain, during May 2018, in order to verify the vulnerability of the aquifers and estimate the possible correlations between surface sources and geochemical anomalies.
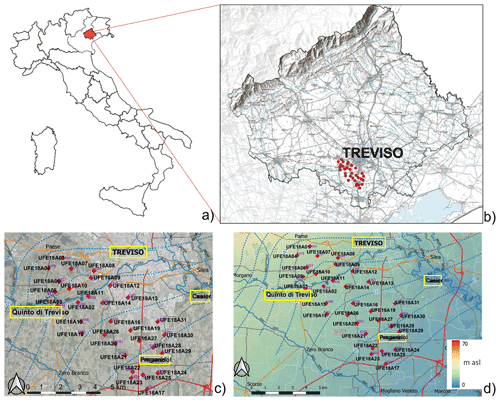
Figure 1Map of the sampling site: (a) Treviso area in the north of Italy coloured in red; (b) location of the sampling wells in the south part of Treviso area; (c) detailed topography map of the sampling wells; (d) detailed hydrogeological map of the sampling wells. All the maps are created using QGIS 3.16 based on the map explained in Mazzola (2003). The four main withdrawal countries are highlighted in light yellow: Quinto di Treviso, Treviso, Preganziol and Casier.
2.1 Sampling area
The sampling area is located in the Venetian Plain (Fig. 1) in the north of Italy (Fig. 1a – coloured in red), a border area between the north-eastern Apennines and the south-eastern Apennines, in the central-southern part of the Middle Plain between the Piave River and the Brenta River (Foraboschi, 2017; Libera et al., 2017).
The area is characterized by a high textural variability of alluvial deposits with different granulometry: at higher heights highly permeable non-consolidated gravel sediments; at sea level terrigenous sequence with prevalent carbonate lithologies; at lower-level clay-silty deposits of environment of coastal area lagoon (Carraro et al., 2015).
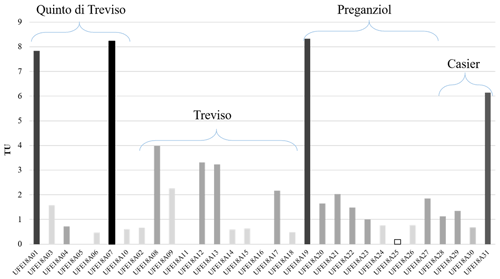
Figure 2Graph showing the different concentrations of tritium (expressed in TU = tritium unit) in the samples collected from the selected wells, separated on the basis of the sampling area: Quinto di Treviso, Treviso, Preganziol and Casier.
Figure 2d shows the detailed hydrogeological map of the sampling site, in which all the wells selected are very closed to sea level. Hydro-geologically, it consists of two units: high and low alluvial plains, separated by a transition zone, known as the resurgence line. The feeding dynamics of the aquifers and the possible interactions at regional and local scale between low salinity freshwater and brackish waters are still little known (Gattacceca et al., 2009; Baruffi et al., 2012; Mayer et al., 2014).
Based on the processing of deep well data, available from the database of the National Mining Office for Hydrocarbons and Georesources (UNMIG) of the Ministry of Economic Development, the SAMPAS project (Area Tecnico Scientifica – Servizio Acque Interne, 2008) highlighted the presence of a morphological depression in the south area of Treviso. This area represents a sector in which the fresh water – salt water interface is located at a depth of about 1000 m, a level higher than that of the neighbouring areas.
Generally, the recharge of the groundwater takes place through river infiltrations (Brenta and Piave rivers), local rains (800–1000 mm yr−1) and irrigation (several million m3 yr−1) or from deep water coming from the carbonate basement of the Venetian Alps and Prealps, where also the waters due to the melting of snowfall flow.
Thanks to the high permeability of gravel sediments, surface waters infiltrate into the sediments recharging the multi-aquifer system of the Venetian Plain. However, this high permeability makes aquifers vulnerable to the diffusion of anthropogenic pollutants that, after a short journey, can spread to various levels in the multi-aquifer system and that can come from:
-
recharge areas of the Superior Plain due to the interaction of surface waters with water contaminated by human activities;
-
water supply of the karst system due to leaching of contaminants from mineralized levels with potentially toxic-harmful metals and from the mining areas that characterize the mountain area of the Venetian Prealps.
2.2 Sampling and analysis techniques
The investigation on tritium concerned the groundwater of 31 selected wells in an area of about 50 km2 (Fig. 1b) that are located in a populated area, as shown in the topography map in Fig. 1c.
The wells were only private water wells in four countries: Quinto di Treviso, Treviso, Preganziol and Casier (highlighted in light yellow in Fig. 2c and d). They were drilled wells with one well screen just at the bottom of the perforation. No pumps were needed because these were only artesian aquifers so water flows under natural pressure without pumping, as it can be seen through the hydrogeological map of the sampling wells in Fig. 2d (Mazzola, 2003).
The waters for the determination of stable isotopes were collected in polyethylene containers, with safety cap and screw cap, with a maximum capacity of 200 mL, while those for tritium in 1 L amber glass containers, with screw cap. The depth of each well (expressed in meters) and the surface elevation (m a.s.l.) are shown in Table 1.
Table 1Tritium concentration (expressed in tritium unit TU) for each sample of water collected, listed for similar sampling site: Quinto di Treviso, Treviso, Preganziol and Casier. In addition, the depth of the wells and the surface elevation (m a.s.l.) are shown (ARPAV report, 2018).
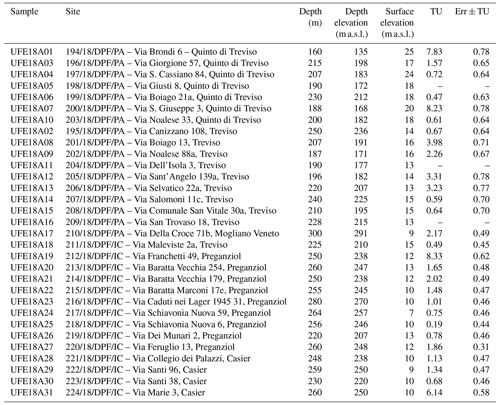
Tritium level in groundwater samples was determined at the Environmental Radiometry Laboratory of the ENEA Research Centre of Brasimone by means of liquid scintillation counting (LSC), as described in the ISO 9698 (2019) and proceeded from an electrolytic enrichment phase in order to reach measurable quantities in the samples. The ENEA laboratory of Brasimone has a very low threshold scintillator LKB QUANTULUS for the determination of 3H in trace (0.2–0.3 TU) and a distillation laboratory and enrichment of aqueous samples for tritium analysis. Electrolytic enrichment was carried out because for the aquifer bodies investigated, the recharge times are rather long and therefore tritium values could be below the detectability threshold for most of the sampled areas (Kaufman and Libby, 1954). For this reason, each sample was subjected to an electrolytic enrichment process prior to measurement. The water sample, after this enrichment, was mixed with a homogeneous and stable scintillating liquid, inserted in a vial into the measuring spectrophotometer. The spectrum obtained is then interpreted thanks to the comparison with reference standards, expressing the intensity of the signal detected in TU concentration. All samples were distilled and concentrated by electrolytic enrichment of water from a maximum volume of 260 mL to about 10 mL. For all the samples, the counting mixture was prepared with 8 mL of sample and 12 mL of scintillating liquid (Ultima Gold LLT, Perkin Elmer, Waltham, Massachusetts, USA) and the sample was measured by liquid scintillation. The average analytical accuracy for tritium is estimated at 0.5 TU (criterion 1σ/analytical errors), which varies for the samples analysed from ±0.31 to ±1.01 TU based on the effective tritium contents.
Isotopic analyses were carried out at the Department of Physics and Earth Sciences of the University of Ferrara. Hydrogen and oxygen isotopic ratios were determined using the CRDS Los Gatos LWIA 24-d isotopic analyser (Marchina et al., 2020). The isotopic ratios of and were expressed as δ notation with respect to the Vienna Standard Mean Ocean Water (V-SMOW) international standard. Three bracketing standards, obtained from the Los Gatos Research Company, were calibrated according to international IAEA (International, Atomic Energy Agency) standards. Analytical precision and accuracy, based on replicate analyses of standards, were better than 0.3 % and 1.0 % for δ18O and δ2H, respectively (Marchina et al., 2019).
In this research paper we calculate the local meteoric water lines (LMWL) that differs from the GMWL (Craig and Gordon, 1965; Crawford et al., 2014) in both slope and deuterium intercept, as a result of varying climatic and geographic parameters (Longinelli and Selmo, 2003; Saccon et al., 2013; Giustini et al., 2016; Paulsson and Widerlund, 2020). The degree by which the LMWL departs from the GMWL can reveal important information about the meteoric sources of water (e.g., oceanic, or terrestrial) and atmospheric conditions during transport (Wang et al., 2018; Boschetti et al., 2019). The regression line between δ2H and δ18O can also be calculated for water samples of different types, such as river waters, soil waters or groundwater (Kaseke et al., 2017; Xia et al., 2020).
In all the wells, the five mandatory parameters required by the Directive 2000/60/EC (2020) must be analysed (dissolved oxygen, pH, electrical conductivity, nitrates, and ammonium ion) and in addition chemical analyses (calcium, magnesium, sodium, potassium, chloride, nitrate, and sulphate) were carried out in the same Laboratory according to standard methods (APHA, 1992), on the waters collected for the determination of stable isotopes. Detection limits are 1 mg L−1 for Na+, Mg2+, Ca2+ and HCO; 0.1 mg L−1 for K+, Cl− and SO; and 0.01 mg L−1 for NO. The results are reported in Table 2. NH and F−, Br−, NO and P− under detection limit.
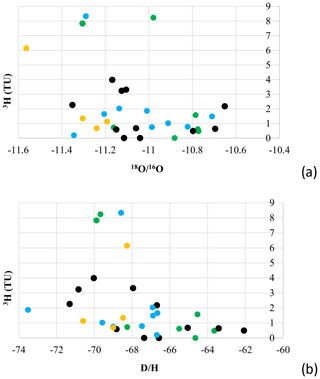
Figure 3Correlation between (a) tritium and δ18O (expressed by the ratio ); (b) tritium and δD (expressed by the ratio ) of each samples analysed. Coloured in green the samples form Quinto di Treviso, in black samples from Treviso, in light blue samples from Preganziol and in yellow samples from Casier.
The term “groundwater age” is still loosely used in literature to denote the time since recharge occurred (Suckow, 2014; Jasechko, 2016; McCallum et al., 2014, 2015; Cartwright et al., 2017). But this implicitly assumes simple non-dispersive, one-dimensional flow (piston flow) where the tracer migrates at a defined velocity, which is almost never the case in reality. Nevertheless, Table 1 and Fig. 2 display the concentrations of tritium for each sample, expressed in TU. All samples show a high concentration of tritium. The samples are classified in five categories:
-
rectangle coloured in white = tritium-free water samples that represent underground water with a long residence time in the subsoil. Tritium in infiltrated waters before 1950 is below the detection threshold since is 12.32 years. These samples characterize aquifers with recharge times over 70 years;
-
rectangle coloured in light grey = water samples with tritium detected with values lower than <0.8 TU but above the detection threshold. These TU values usually concern water with an estimated age of around 50 years (Clark and Fritz, 1997), but considering that from 1952 to 1963 rainfall recorded an increase in tritium, due to nuclear events in the atmosphere, it is now possible to detect tritium even in underground waters with residence time in the subsoil of 70 years. These samples therefore characterize long-recharge aquifers with estimated ages between 50 and 70 years (between 1969 and 1952);
-
rectangle coloured in grey = water samples with 0.8 TU < tritium < 5 TU, whose estimated age is between 10 and 50 years;
-
rectangle coloured in dark grey = water samples with 5 TU < tritium < 7 TU, whose age is estimated between 1 and 10 years (recent recharge);
-
rectangle coloured in black = water samples with 7 TU < tritium < 15 TU, affected by the possible presence of water contamination phenomena (external sources of tritium); it should be kept in mind, however, perturbations from the arctic area (bora wind) can be characterized by an isotopic footprint that reflects the injection of stratospheric air masses with higher tritium content (due to the greater cosmogenic production at the poles) and with very low water vapour content in this very cold air. Consequently, the snow can show an enrichment in tritium and in turn it can be found on the aquifers it feeds. The concentration of tritium, however, can rarely exceed, for the single event of precipitation, the value of 9 TU. Considering that recharging mixes precipitation waters of various origins, this produces a less enriched isotopic footprint.
To better understand the relationships between tritium concentrations in the different wells, tritium values are correlated with the results obtained for stable isotopes (deuterium, oxygen-16 and oxygen-18) in the same samples. The value obtained for the ratio between and the ratio between are shown in Table 2. The sample with the highest concentration of tritium is characterized by an impoverished value of δ18O and δD (Fig. 3). The samples collected in Casier area are generally characterized by low value of and respect most of the other samples ( between −68.2651 and −70.5881; between −11.1918 and −11.5628). Instead of, for the other area, no significant results are observed especially for the diagram in Fig. 3a. Figure 3b shows samples collected in Preganziol area, whose data are more closed to each other respect to the other samples, except for the sample UFE18A19 with high tritium value (8.33 TU).
Chemical data analyses and physic-chemical parameter as temperature (∘C), pH, dissolved oxygen (DO – mg L−1), redox potential (Eh – mV) and electrical conductivity (CE – µS cm−1) are shown in Table 2. Temperature and pH value are generally the same in all the samples analysed: T range between 14.2 and 18.1 ∘C; pH range between 7.5 and 8. CE value change in a range between 362 (in UFE18A24 sample) and 663 µS cm−1 (in UFE18A02 sample), but no specific variation is observed in each sampling site, only in Casier CE value is lower respect to the other areas. The same is for the DO value, that varies in a range between 1.6 and 6.2 mg L−1. On the other hand, different values are shown for Eh value, in which a lot of samples show negative value, instead of the other samples show high positive value, but no specific variation is observed in each sampling site.
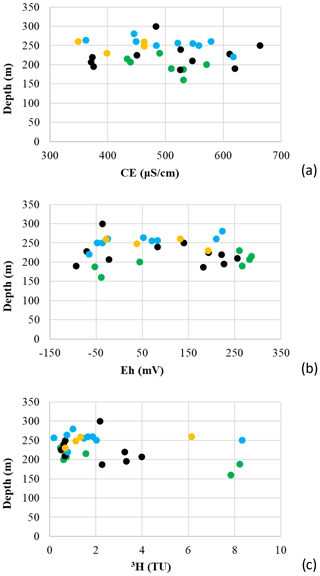
Figure 4Correlation between (a) wells depth (m) and electrical conductivity (µS cm−1); (b) wells depth (m) and redox potential (mV); (c) wells depth (m) and 3H value (TU) of each samples analysed. Coloured in green the samples form Quinto di Treviso, in black samples from Treviso, in light blue samples from Preganziol and in yellow samples from Casier.
The CE and Eh data of each water samples analyzed are compared to the depth of each sampling wells. The results are shown in the graphs of Fig. 4, in which no difference in CE (Fig. 4a) or Eh (Fig. 4b) are observed, but only in the depth of the wells: the data collected in Quinto di Treviso show lower depth value of the wells; on the opposite, Preganziol and Casier show higher value of depth of the wells. No correlation was also observed between the depth of the wells and the tritium value, as shown in Fig. 4c.
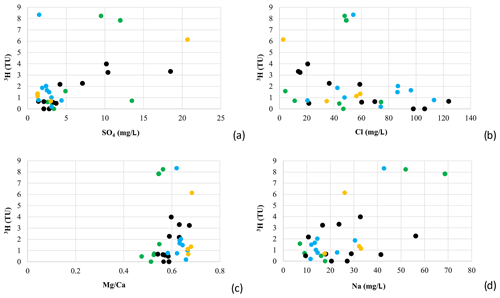
Figure 5Correlation expressed in mg L−1 between tritium and (a) sulphate; (b) chlorides; (c) ratio ; (d) sodium. Coloured in green the samples form Quinto di Treviso, in black samples from Treviso, in light blue samples from Preganziol and in yellow samples from Casier.
For both sulphates and chlorides, no correlations are noted with the results obtained for tritium analyses in the water samples (Fig. 5a and b respectively). It can be seen that the samples form Casier and Preganziol are very closed to each other with low value of SO4 (Preganziol range between 1.4–4.4 mg L−1 and Casier range between 1.3–3 mg L−1 excluding the sample UFE18A31). The sample UFE18A31 collected in Casier area is characterized by high value of tritium (6.14 TU) and high value of SO4 (20.7 mg L−1). No correlation between tritium and SO4 in the samples collected in Treviso and Quinto di Treviso areas.
Instead of, no correlation between tritium and chlorine in the different area analysed are observed (Fig. 5b).
Figure 5c shows the correlation between tritium and the ratio , in which all the samples are very closed to each other, in a range between 0.47 and 0.68.
The diagram 3H-Na in Fig. 5d shows that the samples collected in Preganziol and Quinto di Treviso are generally similar in concentration, except for the samples in which tritium is higher. On the other hands, the samples collected in Treviso and Casier show any correlation.
The geochemical evolution of groundwater can be understood by plotting the concentrations of major cations and anions in the Piper trilinear diagram, using HydroOffice software (Fig. 6).
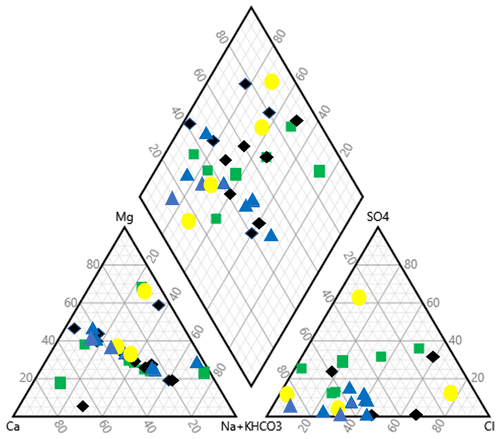
Figure 6Piper diagram for the data collected in the sampling site, using HydroOffice software. Coloured in green the samples form Quinto di Treviso, in black samples from Treviso, in light blue samples from Preganziol and in yellow samples from Casier.
The Preganziol samples (colored in blue in the diagram) are the most homogeneous with respect to the samples collected in the other three locations. In fact, in the two triangles and in the rhombus of the Piper diagram, all the samples are grouped together, representing waters not enriched in Ca and Mg, but located in the sulphate-chlorate sodium-potassium facies (Karmegam et al., 2011).
In Casier's samples (colored in yellow in the diagram of Fig. 6), two groups can be distinguished, each consisting of two samples:
-
2 samples are rich in Ca and Mg: superimposed in the triangle Ca-Mg-Na, located in the upper part of the rhombus diagram; one of these is rich in sulphates, the other in chlorides;
-
2 samples are rich in Na and K and located in the lower part of the rhombus diagram.
As for the samples collected in the wells of Quinto di Treviso (colored in green ion the diagram), the plot shows that most of the samples are mixed in alkaline earths (calcium, sodium, and magnesium) and other samples show similar trend as the samples collected in Preganziol.
The Piper diagram shows that there is no homogeneity between the wells of the different sampling sites and there is not even homogeneity between the wells of Treviso, the data are scattered.
In addition, the results obtained from the collected samples do not show a substantial deviation from the Global Meteoric Water Line (GMWL) and from the Local Meteorological Water Line (LMWL), as shown in Fig. 7.
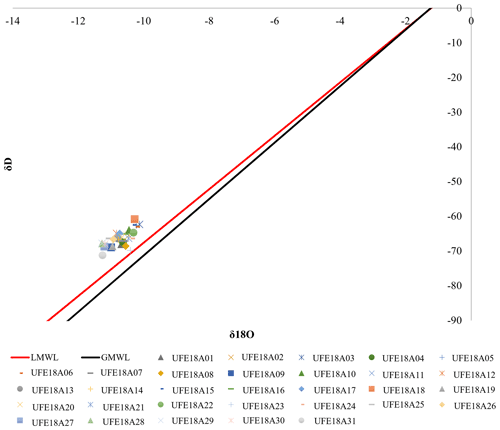
Figure 7Isotopic composition of waters in the sampling collected. Meteoric water lines, global (GMWL – black line) and local (LMWL – red line), were also reported.
In autumn (October 2018) a second campaign was carried out to evaluate any differences in tritium values in the water collected in the same wells compared to the previous campaign. The results of the two campaigns are compared in Fig. 8.
The maximum values of the concentrations of tritium sampled in the autumn period shows values lower than 5 TU and only two samples (UFE18A17 in Treviso and UFE18A21 in Preganziol) are below the detection limit.
The deep-water samples collected in the wells of Preganziol and Casier show higher tritium level in spring than in autumn.
The case of the samples collected in Treviso and Quinto di Treviso wells is different: out of 11 wells monitored in Treviso, in 9 of these the tritium concentration is higher in autumn than in spring. Similar is the case study of Quinto di Treviso, in where out of 6 wells monitored, in 4 of these the tritium concentration is higher in autumn than in spring.
In addition, in all the four sampling sites, the wells in where high level of tritium is observed in spring, also in autumn has the same trend: UFE18A01 and UFE18A07 for Quinto di Treviso; UFE18A08, UFE18A09, UFE18A12 and UFE18A13 for Treviso city; UFE18A19 for Preganziol; UFE18A31 for Casier.
The analyses carried out on the samples of Treviso area show that all the water samples analysed, even those with high TU values, have a low radioactivity and do not exceed the limits established by the Italian legislation (Legislative Decree 212, 2017), which defines in 100 Bq L−1 the limiting activity concentration of tritium (1 TU = 0.119 Bq L−1).
The estimates of age take into account the fact that the content of tritium in groundwater is comparable to that in rainwater. The use of tritium as tracers of young groundwater was also confirmed by different research paper in literature (Nir, 1964; Jurgens et al., 2012; Lindsey et al., 2019), which classified the groundwater age used the assessment and understanding of groundwater resources based on tritium analyses.
Nevertheless, the presence of tritium in groundwater with values higher than those found in rainwater could be due to external contributions, such as leachate from landfills, which host auto-luminescent objects like luminescent paints, displays, light sources and GTLSs/GTLDs (Gaseous Tritium Lights Sources/Devices), night road signs, etc. or from hospital wastewaters or from biological laboratories as tritium thymidine, which is a tracer used in microbiology laboratories (Tazioli et al., 2002; Tazioli, 2011). Ramaroson et al. (2018) shown the extension of groundwater pollution downstream in Madagascar using tritium and stable isotopes (δ2H, δ18O) analyses. Results showed high tritium activities probably of artificial origin.
Since the average data obtained from the Locarno station (GNIP network) show, for the month of March, (average 2000–2009) precipitation with tritium values ranging from a minimum of about 5 TU to a maximum of about 7 TU. It can be said that values lower than 7 TU can reflect a very fast refill, while values higher than 7 denote probable contaminations from surface waters that have interacted with anthropic sources.
Possible contamination phenomena can therefore concern the samples with >7 TU: the presence of tritium contents significantly higher than those characterizing natural waters, suggest that this well have been subject to the interaction with surface waters that have mixed, in their path, with water coming into contact with landfill leachates. However, since there were only 3 wells with this concentration of tritium, this could indicate that the recharge of aquifer systems through contaminated surface waters was low.
As in our case, Jasechko et al. (2017) analyse a lot of wells that are dominated by fossil groundwater also contain detectable levels of tritium, indicating the presence of much younger, decadal-age waters and suggesting that contemporary contaminants may be able to reach deep wells that tap fossil aquifers. The same for Cook et al. (2018), that demonstrated that in most natural systems porewater exchange and groundwater inflow occur concurrently. The use of tracers, and spatial and temporal replication should provide a more complete picture of exchange processes and the extent of subsurface mixing. Also, Zhao et al. (2018) used stable hydrogen and oxygen isotopes to investigate the interaction between groundwater and surface water along two rivers in the northwest China observing corresponding changes along these two rivers, which also provided evidence for the groundwater-surface water interactions.
Regarding our research work, additional information on the anthropogenic interaction were observed. The tritium values for some wells were higher than those characterizing rainwater in the order of a few percentage points, this probably means possible interactions with landfill leachate (IAEA/UNESCO, 2020). Knowing that the rate of diffusion of tritium is major than all the other types of pollutants, this methodology is a useful approach to highlight in advance a plume of inorganic and organic chemical pollutants that can compromise the aquifer in the future. It would therefore be interesting to extend the tritium analysis to neighbouring wells in order to better define the plume and map its origin.
In addition, isotope analyses allow us to fully respond to the purposes of the legislation relating to environmental protection (Legislative Decree 152, 2006) in particular to water resources following the Directive 2013/51/EURATOM (2013).
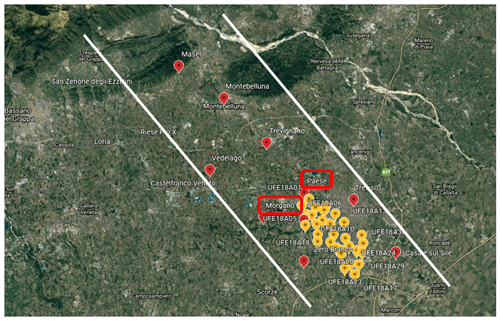
Figure 9Map obtained by © Google Earth of the wells sampling site coloured in yellow and coloured in red the sampling area of the underground water analysed by ARPAV. Squared in red the towns of Paese and Morgano very closed to sampling analysed in this paper.
Considering the data published by ARPAV of the last ten years on water quality (ARPAV, 2020), and taking into consideration only the data of the sampling sites close to the wells analysed, it can be seen that in some years the waters related to the towns of Paese and Morgano (marked in red on the map in Fig. 9) have not always been in good condition. High concentrations of nitrates have often been found (ARPAV report, 2011, 2012, 2013, 2014). In the ARPAV report related to the 2018-year, period of sampling analysed in this paper, the ARPAV data are all good quality without highlighting particular anomalies. This, of course, is not in line with the data obtained with tritium analyses, which instead show considerable differences probably related to the underground geomorphology of the sampling site.
The whole sampling area is vulnerable to contamination of pollutants that may come from: recharge areas of the Upper Plain for the interaction of surface waters with water contaminated by anthropogenic activities; water contributions from the karst system due to the leaching of contaminants from mineralized levels with potentially toxic metals and from the mining areas that characterize the mountainous area of the Venetian Pre-Alps.
Based on the processing of data from deep wells, the SAMPAS project (Area Tecnico Scientifica – Servizio Acque Interne, 2008) highlighted the presence of a morphological depression in the south area of Treviso, in which the freshwater – saltwater interface is located at the depth of about 1000 m, a level higher than that of the neighboring areas. This depression has favored, in the periods of marine ingression, the formation of pelitic sedimentation lagoons interspersed with sands referable to lagoon systems that evolve with dune strands towards marine conditions (Bondesan and Meneghel, 2004) that can host fossil salt waters.
The high permeability of the high plain allows surface waters to infiltrate, thus representing the recharge area of the water bodies of the multi-aquifer system of the Venetian Plain. The high permeability of gravelly sediments makes groundwater aquifers vulnerable to the spread of anthropogenic pollutants which, after a short journey, can spread to various levels in the multi-aquifer system, the vulnerability transfers, in fact, to the semi-confined and confined system fed mainly from the contributions of surface waters from the high plain. As well as from surface waters, recharging can also take place from contributions of deep water from the carbonate basement fed, in turn, by the carbonate system of the Venetian Alps and Prealps, into which the melting waters of snowfall also flow. The recharge of this water table takes place through river infiltrations (Brenta and Piave rivers), local rainfall (800–1000 mm a−1) and irrigation (several million m3 a−1).
For all these reasons, it is more important to better characterize the geochemical fingerprint of each well to identify the water recharge contributions and possible markers from the interaction between water and sediments (e.g., chlorine, sodium…).
Future work will be implemented with all these data especially regarding the precipitation water tritium concentrations that will give us additional important information regarding the interaction of rainwater into the aquifer.
In this research work, the analyzes showed an increase in chlorides and electrical conductivity in the water which could indicate salinization processes (Paine, 2003), however referable to contexts of fossil waters in aquifers and not to the intrusion of the saline wedge, as the is not characteristic of the sea water and also the evolutionary trends of trace metal compositions are not correlated with a mixing trend. Rahal et al. (2021) indicated that the groundwater resource is suffering from salinization, mainly due to evaporation and leaching of soil salts, a process that is coupled with simultaneous cation-exchange effects. In our work this could be due to the chloride ions presence that could interact with the soil of the aquifer. In addition, the isotopic footprint of this monitoring campaign is positioned on the GMWL meteoric line, in accordance with the age of the recharge waters detected through the analysis of the tritium concentration for most of the wells. The aquifer in the Treviso plain area is, therefore, extremely vulnerable to climate change and in particular to prolonged drought periods, which could induce salinization dynamics. Salinization is due to long periods of permanence of the water in the aquifer which favors the water-rock interaction (Walter et al., 2017; M'Nassri et al., 2019). The interactions produce, in fact, a worsening of water quality, consequently limiting water availability (Álvarez-Cobelas et al., 2010).
Finally, tritium analyses allow to verify the presence of strong differences in recharge by recent rainwater (Mahlangu et al., 2020), which reflect the change in permeability of the gravelly sediments of the High and Middle Plains compared to the less permeable sediments of the transition area of the springs. The results obtained show a strong variability in the tritium units and that in only a few wells there are indicative values of recent recharge. These vulnerable contexts require more attention on the possible contributions of surface water that may have interacted with anthropogenic components (pollution).
Remembering that tritium decays exponentially, common analysis techniques do not allow the determination of tritium in bodies older than twenty to thirty years. Consequently, if there are contributions of surface water in fossil aquifers, even low percentages of recharge modify the concentration of tritium and if there are anthropogenic contributions, tritium values higher than the natural thresholds can be observed.
In order to date the aquifers, it is therefore necessary to use enrichment strategies that allow the determination of tritium even in very low concentration. For this study, since the aquifers were investigated deep bodies with low recharge, we used the method of distillation and electrolytic enrichment and subsequent analysis in liquid scintillation with success. The results highlighted differences in abundances that reflect possible interactions between fossil waters and surface recharge inputs. Only on four samples the current contribution is strongly evident, while in many other samples there are low values which may indicate either more or less long recharge times and/or low recharge in fossil aquifers induced by over-exploitation. The data limited to a single monitoring campaign provide a temporal examination and only analyzes repeated over time and in various drought conditions can allow us to discriminate which of the two hypotheses is realistic. Analyzing the concentration of tritium in two different and opposite seasons (spring and autumn), it is possible to identify important differences that highlight the different contributions of surface water. Future analyzes in the same seasons and at other times of the year will be able to provide a broad spectrum of the real situation of the aquifer examined.
Considering that even insignificant volumes of recent tritium water can modify the tritium footprint from fossil aquifers, this research work through the monitoring of the tritium content can allow to determine the vulnerability of the aquifer with a high confidence in the results and, therefore, to provide a useful tool to correlate the sources of pollution with the possible geochemical anomalies observed (Abdullah et al., 2018), to define targeted protection plans to preserve strategic freshwater fossil resources from potential risks (Pradinaud et al., 2019) and to identify the best practices for the protection of the aquifers (Ficco and Sasowsky, 2018). The identification of non-polluted freshwater free of tritium to be protected for future generations could become part of this perspective.
Tritium concentration analyses were performed on selected samples of groundwater collected in different wells in the south part of Treviso city. The high presence and concentration of this radionuclide is correlated with the young age of the water recharge.
The results made it possible to verify, thanks to the tritium analysis, strong differences in recharge by recent rainwater which reflect the variation in permeability of the gravelly sediments of the Upper and Middle Plains compared to the less permeable sediments of the resurgence transition area. The abundance of young waters indicates a vulnerability with respect to possible contributions of surface waters.
The samples, in fact, had an isotopic imprint that was positioned on the meteoric line in accordance with the young age of the recharge waters detected through tritium analyses for most of the wells.
The aquifer in the Treviso plain area was therefore extremely vulnerable to climate change and in particular to prolonged drought periods which could induce salinization dynamics (especially shown in the spring sampling). Salinization, generally, is due to long periods of permanence of the water in the aquifer which favours the water-rock interaction. The interactions produce a worsening of water quality, consequently limiting water availability.
This research work allowed to better know the hydrogeochemical characteristics of the aquifers in the pedemontana area of the Venetian plain and to better understanding the presence of the phenomena of contamination by anthropogenic activities, harmful to human health.
It will be necessary to carry out several and repeated measurement campaigns to constantly monitor the aquifer in the Treviso area to better understand the recharge of the aquifer and prevent possible pollution from outside.
The software used were Microsoft Office and QGIS 3.16.
All the data used to obtain figures and graphs are described in the Tables in the manuscript.
AP and CV identified the scope of this work; CT, AP, AR, SS, EM and CV designed the experiments and SS carried them out. CT, AP and EM elaborated the data. CT prepared the manuscript with contributions from all co-authors.
The contact author has declared that neither they nor their co-authors have any competing interests.
Publisher's note: Copernicus Publications remains neutral with regard to jurisdictional claims in published maps and institutional affiliations.
This article is part of the special issue “Geoscience applications of environmental radioactivity (EGU21 GI6.2 session)”. It is a result of the EGU General Assembly 2021, 19–30 April 2021.
This paper was edited by Virginia Strati and reviewed by three anonymous referees.
Abdullah, T. O., Ali, S. S., Al-Ansari, N. A., and Knutsson, S.: Possibility of Groundwater Pollution in Halabja Saidsadiq Hydrogeological Basin, Iraq Using Modified DRASTIC Model Based on AHP and Tritium Isotopes, Geosciences, 8, 236, https://doi.org/10.3390/geosciences8070236, 2018.
Ala-aho, P., Soulsby, C., Pokrovsky, O. S., Kirpotin, S. N., Karlsson, J., Serikova, S., Vorobyev, S. N., Manasypov, R. M., Loiko, S., and Tetzlaff, D.: Using stable isotopes to assess surface water source dynamics and hydrological connectivity in a high-latitude wetland and permafrost influenced landscape, J. Hydrol., 556, 279–293, https://doi.org/10.1016/j.jhydrol.2017.11.024, 2018.
Álvarez-Cobelas, M., Sánchez-Carrillo, S., Cirujano, S., and Angeler, D. G.: A Story of the Wetland Water Quality Deterioration: Salinization, Pollution, Eutrophication and Siltation, in: Ecology of Threatened Semi-Arid Wetlands. Wetlands: Ecology, Conservation and Management, 2, edited by: Sánchez-Carrillo, S. and Angeler, D., Springer, Dordrecht, https://doi.org/10.1007/978-90-481-9181-9_5, 2010.
APHA: Standard Methods for Water and Wastewater Examination, 17th edn., edited by: Greenberg, A. E., Clescerl, L. S., and Eaton, A. D., American Public Health Association, Washington, D.C., USA, 1992.
Area Tecnico Scientifica – Servizio Acque Interne: Le acque sotterranee della pianura veneta – I risultati del Progetto SAMPAS, Orientambiente, 1–104, 2008.
ARPAV: Qualità delle acque sotterranee 2019, ARPA VENETO, 2020.
ARPAV report: https://www.arpa.veneto.it/dati-ambientali/open-data/idrosfera/acque-sotterranee/acque-sotterranee-qualita-chimica (last access: 15 September 2021), 2011, 2012, 2013, 2014.
ARPAV report: Monitoraggio delle acque sotterranee in alcuni comuni della provincia di Treviso per il controllo dell'inquinamento da mercurio (Hg), ARPAV, Unità Operativa Qualità acque Interne, edited by: Moretto, C. G. and Boscolo, C., 2018.
Baruffi, F., Cisotto, A., Cimolino, A., Ferri, M., Monego, M., Norbiato, D., Cappelletto, M., Bisaglia, M., Pretner, A., Galli, A., Scarinci, A., Marsala, V., Panelli, C., Gualdi, S., Bucchignani, E., Torresan, S., Pasini, S., Critto, A., and Marcomini, A.: Climate change impact assessment on Veneto and Friuli plain groundwater. Part I: An integrated modelling approach for hazard scenario construction, Sci. Total Environ., 440, 154–166, https://doi.org/10.1016/j.scitotenv.2012.07.070, 2012.
Bondesan, A. and Meneghel, M.: Geomorfologia della provincia di Venezia, Padova, Esedra, 516, 2004.
Boral, S., Sen, I. S., Ghoshal, D., Peucker-Ehrenbrink, B., and Hemingway, J.: Stable water isotope modeling reveals Spatio-temporal variability of glacier meltwater contributions to Ganges River headwaters, J. Hydrol., 577, 123983, https://doi.org/10.1016/j.jhydrol.2019.123983, 2019.
Boschetti, T., Cifuentes, J., Iacumin, P., and Selmo, E.: Local Meteoric Water Line of Northern Chile (18∘ S–30∘ S): An Application of Error-in-Variables Regression to the Oxygen and Hydrogen Stable Isotope Ratio of Precipitation, Water, 11, 791, https://doi.org/10.3390/w11040791, 2019.
Bronić, K. I. and Barešić, J.: Application of Stable Isotopes and Tritium in Hydrology, Water, 13, 430, https://doi.org/10.3390/w13040430, 2021.
Bryan, E., Meredith, K. T., Baker, A., Post, V. E. A., and Andersen, M. S.: Island groundwater resources, impacts of abstraction and a drying climate: Rottnest Island, Western Australia, J. Hydrol., 542, 704–718, https://doi.org/10.1051/e3sconf/20185400022, 2016.
Carraro, A., Fabbri, P., Giaretta, A., Peruzzo, L., Tateo, F., and Tellini, F.: Effects of redox conditions on the control of arsenic mobility in shallow alluvial aquifers on the Venetian Plain (Italy), Sci. Total Environ., 532, 581–594, https://doi.org/10.1016/j.scitotenv.2015.06.003, 2015.
Cartwright, I., Cendón, D., Currell, M., and Meredith, K.: A review of radioactive isotopes and other residence time tracers in understanding groundwater recharge: Possibilities, challenges, and limitations, J. Hydrol., 555, 797–811, https://doi.org/10.1016/j.jhydrol.2017.10.053, 2017.
Cauquoin, A., Jean-Baptiste, P., Risi, C., Fourré, É., Stenni, B., and Landais A.: The global distribution of natural tritium in precipitation simulated with an Atmospheric General Circulation Model and comparison with observations, Earth Planet. Sci. Lett., 427, 160–170, https://doi.org/10.1016/j.epsl.2015.06.043, 2015.
Chen, K., Meng, Y., Liu, G., Xia, C., Zhou, J., and Li, H.: Identifying hydrological conditions of the Pihe River catchment in the Chengdu Plain based on spatio-temporal distribution of 2H and 18O, J. Radioanal. Nuc. Chem., 324, 1125–1140, https://doi.org/10.1007/s10967-020-07163-z, 2020.
Clark, I. and Fritz, P.: Environmental Isotopes in Hydrogeology, Lewis Book, 1st edn., ISBN 1-56670-249-6, 1997.
Cook, P. G., Rodellas, V., and Stieglitz, T. C.: Quantifying surface water, porewater, and groundwater interactions using tracers: Tracer fluxes, water fluxes, and end-member concentrations, Water Resour. Res., 54, 2452–2465, https://doi.org/10.1002/2017WR021780, 2018.
Craig, H. and Gordon, L. I.: Deuterium and oxygen-18 variations in the ocean and the marine atmosphere, in: Stable Isotopes in Oceanographic Studies and Palaeotemperatures, edited by: Tongiorgi, E., Laboratorio di geologia nucleare, Spoleto, Italy, 9–130, 1965.
Crawford, J., Hughes, C. E., and Lykoudis, S.: Alternative least squares methods for determining the meteoric water line, demonstrated using GNIP data, J. Hydrol., 519, 2331–2340, https://doi.org/10.1016/j.jhydrol.2014.10.033, 2014.
Directive 2000/60/EC: Framework for Community action in the field of water policy, Official Journal, L327, 0001–0073, 2020.
Directive 2013/51/EURATOM: Requirements for the protection of the health of the general public with regard to radioactive substances in water intended for human consumption, http://data.europa.eu/eli/dir/2013/51/oj (last access: 15 September 2021), 2013.
DOE Handobook: Tritium handling and safe storage, U.S. Department of Energy, Washington, D.C., 20585, 2008.
Ehhalt, D. H., Rohrer, F., Schauffler, S., and Pollock, W.: Tritiated water vapor in the stratosphere: vertical profiles and residence time, J. Geophys. Res., 107, 4757, https://doi.org/10.1029/2001JD001343, 2002.
Evaristo, J., McDonnell, J. J., and Clemens, J.: Plant source water apportionment using stable isotopes: A comparison of simple linear, two-compartment mixing model approaches, Hydrol. Process., 31, 3750–3758, https://doi.org/10.1002/hyp.11233, 2017.
Ficco, K. K. and Sasowsky, I. D.: An interdisciplinary framework for the protection of karst aquifers, Environ. Sci. Policy, 89, 41–48, https://doi.org/10.1016/j.envsci.2018.07.005, 2018.
Foraboschi, P.: Specific structural mechanics that underpinned the construction of Venice and dictated venetian architecture, Eng. Fail. Anal., 78, 169–195, https://doi.org/10.1016/j.engfailanal.2017.03.004, 2017.
Fourré, E., Jean-Baptiste, P., Dapoigny, A., Baumier, D., Petit, J. R., and Jouzel, J.: Past and recent tritium levels in Arctic and Antarctic polar caps, Earth Planet. Sci. Lett., 245, 56–64, https://doi.org/10.1016/j.epsl.2006.03.003, 2006.
Gattacceca, J. C., Vallet-Coulomb, C., Mayer, A., Claude, C., Radakovitch, O., Conchetto, E., and Hamelin, B.: Isotopic and geochemical characterization of salinization in the shallow aquifers of a reclaimed subsiding zone: The southern Venice Lagoon coastland, J. Hydrol., 378, 46–61, https://doi.org/10.1016/j.jhydrol.2009.09.005, 2009.
Gibson, J. J., Holmes, T., Stadnyk, T. A., Birks, S. J., Eby, P., and Pietrniro, A.: 18O and 2H in streamflow across Canada, J. Hydrol., 32, 100754, https://doi.org/10.1016/j.ejrh.2020.100754, 2020.
Giustini, F., Brilli, M., and Patera, A.: Mapping oxygen stable isotopes of precipitation in Italy, J. Hydrol. Reg. Stud., 8, 162–181, https://doi.org/10.1016/j.ejrh.2016.04.001, 2016.
Glibert, P. M., Middelburg, J. J., McClelland, J. W., and Jake Vander Zanden, M.: Stable isotope tracers: Enriching our perspectives and questions on sources, fates, rates, and pathways of major elements in aquatic systems, Limnol. Oceanogr., 64, 950–981, https://doi.org/10.1002/lno.11087, 2019.
Guo, C., Shi, J., Zhang, Z., and Zhang, F.: Using tritium and radiocarbon to determine groundwater age and delineate the flow regime in the Taiyuan Basin, China, Arab. J. Geosci., 12, 185, https://doi.org/10.1007/s12517-019-4371-7, 2019.
IAEA/WMO: Global Network of Isotopes in Precipitation, The GNIP Database, IAEA, http://www.iaea.org/water (last access: 15 September 2021), 2019.
ISO 9698: Water quality – Tritium – Test method using liquid scintillation counting, BSI Standard Publications, 2019.
Jasechko, S.: Partitioning young and old groundwater with geochemical tracers, Chem. Geol., 427, 35–42, https://doi.org/10.1016/j.chemgeo.2016.02.012, 2016.
Jasechko, S., Perrone, D., Befus, K., Cardenas, M. B., Ferguson, G., Gleeson, T., Luijendijk, E., McDonnell, J. J., Taylor, R. G., Wada, Y., and Kirchner, J. W.: Global aquifers dominated by fossil groundwaters but wells vulnerable to modern contamination, Nat. Geosci., 10, 425–429, https://doi.org/10.1038/ngeo2943, 2017.
Jerbi, H., Hamdi, M., Snoussi, M., Abdelmalek, M. B., Jnoub, H. and Tarhouni, J.: Usefulness of historical measurements of tritium content in groundwater for recharge assessment in semi-arid regions: application to several aquifers in central Tunisia, Hydrogeol. J., 27, 1645–1660, https://doi.org/10.1007/s10040-019-01937-w, 2019.
Jurgens, B. C., Böhlke, J. K., and Eberts, S. M.: TracerLPM (Version 1): An Excel® Workbook for Interpreting Groundwater Age Distributions from Environmental Tracer Data, USGS, 2012.
Kaseke, K. F., Wang, L., and Seely, M. K.: Nonrainfall water origins and formation mechanisms, Sci. Adv., 3, e1603131, https://doi.org/10.1126/sciadv.1603131, 2017.
Karmegam, U., Chidambaram, S., Prasanna, M. V., Sasidhar, P., Manikandan, S., Johnsonbabu, G., Dheivanayaki, V., Paramaguru, P., Manivannan, R., Srinivasamoorthy, K., and Anandhan, P.: A study on the mixing proportion in groundwater samples by using Piper diagram and Phreeqc model, Chin. J. Geochem., 30, 490, https://doi.org/10.1007/s11631-011-0533-3, 2011.
Kaufman, S. and Libby, W. F.: The natural distribution of tritium, Phys. Rev., 93, 1337–1344, https://doi.org/10.1103/PhysRev.93.1337, 1954.
Kim, H., Cho, S. H., Lee, D., Jung, Y. Y., Kim, Y. H., Koh, D. C., and Lee, J.: Influence of pre-event water on streamflow in a granitic watershed using hydrograph separation, Environ. Earth Sci., 76, 82, https://doi.org/10.1007/s12665-017-6402-6, 2017.
Legislative Decree 152: Norme in materia ambientale, Gazzetta Ufficiale, 88, 2006.
Legislative Decree 212: Indicazioni operative a carattere tecnico-scientifico, ai sensi dell'articolo 8 del decreto legislativo 15 febbraio 2016, Gazzetta Ufficiale, 28, 2017.
Libera, N. D., Fabbri, P., Mason, L., Piccinini, L., and Pola, M.: Geostatistics as a tool to improve the natural background level denition: An application in groundwater, Sci. Total Environ., 598, 330–340, https://doi.org/10.1016/j.scitotenv.2017.04.018, 217.
Lindsey, B. D., Jurgens, B. C., and Belitz, K.: Tritium as an indicator of modern, mixed, and premodern groundwater age, Bulletin: Scientific Investigations Report – US Geological Survey 2019 No. 2019-5090 pp.vii + 18 pp., ref. 49, 2019.
Longinelli, A. and Selmo, E.: Isotopic composition of precipitation in Italy: a first overall map, J. Hydrol., 270, 75–88, https://doi.org/10.1016/S0022-1694(02)00281-0, 2003.
M'Nassri, S., Lucas, Y., Schäfer, G., Dridi, L., and Majdoub, R.: Coupled hydrogeochemical modelling using KIRMAT to assess water-rock interaction in a saline aquifer in central-eastern Tunisia, Appl. Geochemistry, 102, 229–242, https://doi.org/10.1016/j.apgeochem.2019.01.018, 2019.
MacMahon, D.: Half-life evaluations for 3H, 90Sr, and 90Y, Appl. Radiat. Isot., 64, 1417–1419, https://doi.org/10.1016/j.apradiso.2006.02.072, 2006.
Mahlangu, S., Lorentz, S., Diamond, R., and Dippenaar, M.: Surface water-groundwater interaction using tritium and stable water isotopes: A case study of Middelburg, South Africa, J. Afr. Earth Sci., 171, 103886, https://doi.org/10.1016/j.jafrearsci.2020.103886, 2020.
Marchina, C., Natali, C., and Bianchini, G.: The Po River Water Isotopes during the Drought Condition of the Year 2017, Water, 11, 150, https://doi.org/10.3390/w11010150, 2019.
Marchina, C., Lencioni, V., Paoli, F., Rizzo, M., and Bianchini, G.: Headwaters' Isotopic Signature as a Tracer of Stream Origins and Climatic Anomalies: Evidence from the Italian Alps in Summer 2018, Water, 12, 390, https://doi.org/10.3390/w12020390, 2020.
Mayer, A., Sültenfuß, J., Travi, Y., Rebeix, R., Purtschert, R., Claude, C., Le Gal La Salle, C., Miche, H., and Conchetto, E.: A multi-tracer study of groundwater origin and transit-time in the aquifers of the Venice region (Italy), Appl. Geochem., 50, 177–198, https://doi.org/10.1016/j.apgeochem.2013.10.009, 2014.
Mazzola, M.: Idrogeologia e carta freatimetrica della Provincia di Treviso, Provincia di Treviso, Assessorato alle politiche per l'Ambiente, 2003.
McCallum, J. L., Engdahl, N. B., Ginn, T. R., and Cook, P. G.: Nonparametric estimation of groundwater residence time distributions: What can environmental tracer data tell us about groundwater residence time?, Water Resour. Res., 50, 2022–2038, https://doi.org/10.1002/2013WR014974, 2014.
McCallum, J. L., Cook, P. G., Simmons, C. T.: Limitations of the Use of Environmental Tracers to Infer Groundwater Age, Groundwater, 53, 56–70, https://doi.org/10.1111/gwat.12237, 2015.
Mello, R. S., Luna, A. S., Ferreira, A. A., Tonietto, G. B., Bittencourt, I., and Godoy J. M. O.: Development and validation of an analytical methodology for the determination of δ2H and δ18O in formation water based on Laser-Based infrared absorption spectroscopy, Microchem. J., 155, 104678, https://doi.org/10.1016/j.microc.2020.104678, 2020.
Morgenstern, U., Stewart, M. K., and Stenger, R.: Dating of streamwater using tritium in a post nuclear bomb pulse world: continuous variation of mean transit time with streamflow, Hydrol. Earth Syst. Sci., 14, 2289–2301, https://doi.org/10.5194/hess-14-2289-2010, 2010.
Morgenstern, U., Daughney, C. J., Leonard, G., Gordon, D., Donath, F. M., and Reeves, R.: Using groundwater age and hydrochemistry to understand sources and dynamics of nutrient contamination through the catchment into Lake Rotorua, New Zealand, Hydrol. Earth Syst. Sci., 19, 803–822, https://doi.org/10.5194/hess-19-803-2015, 2015.
Nir, A.: On the interpretation of tritium `age' measurements of groundwater, J. Geophys. Res., 69, 2589–2595, https://doi.org/10.1029/JZ069i012p02589, 1964.
Paine, J. G.: Determining salinization extent, identifying salinity sources, and estimating chloride mass using surface, borehole, and airborne electromagnetic induction methods, Water Resour. Res., 39, 1059, https://doi.org/10.1029/2001WR000710, 2003.
Paulsson, O. and Widerlund, A.: Pit lake oxygen and hydrogen isotopic composition in subarctic Sweden: A comparison to the local meteoric water line, Appl. Geochem., 118, 104611, https://doi.org/10.1016/j.apgeochem.2020.104611, 2020.
Pradinaud, C., Northey, S., Amor, B., Bare, J., Benini, L., Berger, M., Boulay, A. M., Junqua, G., Lathuillière, M. J., Margni, m., Motoshita, M., Niblick, B., Payen, S., Pfister, S., Quinteiro, P., Sonderegger, T., and Rosenbaum, R. K.: Defining freshwater as a natural resource: a framework linking water use to the area of protection natural resources, Int. J. Life Cycle Assess. 24, 960–974, https://doi.org/10.1007/s11367-018-1543-8, 2019.
Rahal, O., Gouaidia, L., Fidelibus, M. D., Marchina, C., Natali, C., and Bianchini, G.: Hydrogeological and geochemical characterization of groundwater in the F'Kirina plain (eastern Algeria), Appl. Geochem., 130, 104983, https://doi.org/10.1016/j.apgeochem.2021.104983, 2021.
Ramaroson, V., Rakotomalala, C. U., Rajaobelison, J., Fareze, L. P., Razafitsalama, F. A., and Rasolofonirina, M.: Tritium as tracer of groundwater pollution extension: case study of Andralanitra landfill site, Antananarivo–Madagascar, Appl. Water Sci., 8, 57, https://doi.org/10.1007/s13201-018-0695-9, 2018.
Rozanski, K., Araguas-Araguas, L., and Gonfiantini, R.: Tritium in the global atmosphere: distribution, patterns and recent trends, J. Phys. G-Nucl. Part. Phys., 17, S523–S536, https://doi.org/10.1088/0954-3899/17/S/053, 1991.
Saccon, P., Leis, A., Marca, A., Kaiser, J., Campisi, L., Böttcher, M. E., Savarino, J., Escher, P., Eisenhauer, A., and Erbland, J.: Multi-isotope approach for the identification and characterisation of nitrate pollution sources in the Marano lagoon (Italy) and parts of its catchment area, Appl. Geochem., 34, 75–89, https://doi.org/10.1016/j.apgeochem.2013.02.007, 2013.
Schmieder, J., Garvelmann, J., Marke, T., and Strasser, U.: Spatiotemporal tracer variability in the glacier melt end-member - How does it affect hydrograph separation results?, Hydrol. Process., 32, 1828–1843, https://doi.org/10.1002/hyp.11628, 2018.
Silva, A. and Cota, S.: Groundwater age dating using single and time-series data of environmental tritium in the Moeda Synclyne, Quadrilátero Ferrífero, Minas Gerais, Brazil, J. South Am. Earth Sci., 107, 103009, https://doi.org/10.1016/j.jsames.2020.103009, 2020.
Suckow, A.: The age of groundwater – Definitions, models and why we do not need this term, Appl. Geochem., 50, 222–230, https://doi.org/10.1016/j.apgeochem.2014.04.016, 2014.
Tao, Z., Li, M., Si, B., and Pratt, D.: Rainfall intensity affects runoff responses in a semi-arid catchment, Hydrol. Process., 35, e14100, https://doi.org/10.1002/hyp.14100, 2021.
Tazioli, A.: Experimental methods for river discharge measurements: comparison among tracers and current meter, Hydrol. Sci. J., 56, 1314–1324, https://doi.org/10.1080/02626667.2011.607822, 2011.
Tazioli, A., Boschi, G., and Carlini, A.: Monitoraggio dell'inquinamento da discariche: metodi isotopici per individuare la presenza di contaminazione delle acque sotterranee, Giorn. Geol. Appl., 2, 130–136, https://doi.org/10.1474/GGA.2005-02.0-19.0045, 2002.
UNSCEAR: Sources and Effects of Ionizing Radiation: Sources, United Nations Publications, 2000.
UNSCEAR: Sources and Effects of Ionizing Radiation: Sources, United Nations Publications, 2016.
Walter, J., Chesnaux, R., Cloutier, V., and Gaboury, D.: The influence of water/rock − water/clay interactions and mixing in the salinization processes of groundwater, J. Hydrol. Reg. Stud., 13, 168–188, https://doi.org/10.1016/j.ejrh.2017.07.004, 2017.
Wang, S., Zhang, M., Hughes, C. E., Crawford, J., Wang, G., Chen, F., Du, M., Qiu, X., and Zhou, S.: Meteoric water lines in arid Central Asia using event-based and monthly data, J. Hydrol., 562, 435–445, https://doi.org/10.1016/j.jhydrol.2018.05.034, 2018.
Xia, C., Liu, G., Meng, Y., Wang, Z., and Zhang, X.: Impact of human activities on urban river system and its implication for water-environment risks: an isotope-based investigation in Chengdu, China, Hum. Ecol. Risk Assess., 27, 1416–1439, https://doi.org/10.1080/10807039.2020.1848416, 2020.
Yang, J., Dudley, B. D., Montgomery, K., and Hodgetts, W.: Characterizing spatial and temporal variation in 18O and 2H content of New Zealand river water for better understanding of hydrologic processes, Hydrol. Process., 34, 5474–5488, https://doi.org/10.1002/hyp.13962, 2020.
Zhao, M., Hu, Y., Zeng, C., Liu, Z., Yang, R., and Chen, B.: Effects of land cover on variations in stable hydrogen and oxygen isotopes in karst groundwater: A comparative study of three karst catchments in Guizhou Province, Southwest China, J. Hydrol., 565, 374–385, https://doi.org/10.1016/j.jhydrol.2018.08.037, 2018.
Zhou, J., Liu, G., Meng, Y., Xia, C. C., Chen, K., and Chen, Y.: Using stable isotopes as tracer to investigate hydrological condition and estimate water residence time in a plain region, Chengdu, China, Sci. Rep.-UK, 11, 2812, https://doi.org/10.1038/s41598-021-82349-3, 2021.