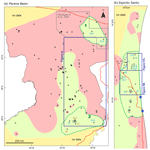
Geological screening of onshore saline aquifers for CO2 storage: Paraná and Espírito Santo basins, Brazil
Francyne Bochi do Amarante
Juliano Kuchle
Claiton Marlon dos Santos Scherer
Shahin E. Dashtgard
Maurício Barcelos Haag
To meet the global goal of net-zero CO2 emissions by 2050, many governments are turning to Carbon Capture and Storage (CCS) as a critical technology. Brazil is the largest CO2 emitter in South America and the 7th largest globally, yet it is in the early stages of CCS development. Using publicly available geological and infrastructure data, this study evaluates the feasibility and suitability of saline aquifers for CO2 storage in onshore intracratonic basins in southern Brazil. Saline aquifers deemed suitable for CO2 sequestration are those below 800 m deep, with a thickness greater than 20 m, and in areas with regionally extensive caprock at least 10 m thick. Using these criteria, we classify areas within the Rio Bonito Formation of the Paraná Basin and the Mucuri Member in the onshore extent of the Espírito Santo Basin into potential and exclusion areas for CO2 storage. The Rio Bonito Formation is present in the central-southern Paraná Basin and ranges from outcrop exposures to over 3900 m depth. The formation ranges in thickness from 25–350 m, and sandstone intervals within the formation have a cumulative thickness between 13 and 150 m. Caprocks consist mainly of shale and siltstone. Based on the criteria used, CO2 sequestration in the Rio Bonito Formation is optimal in two zones of the Paraná Basin that extend over ca. 27 700 km2 (northern zone) and ca. 38 100 km2 (southern zone). The Mucuri Member is present in almost the entire onshore extent of the Espírito Santo Basin and ranges in depth from 500 to 2650 m. The unit reaches 400 m thick with a cumulative thickness of sandstone layers ranging from 0 to 320 m. Caprocks consist mainly of evaporites, but shale and sandstone intervals are also present. CO2 sequestration in the Mucuri Member is optimal in one zone of the onshore Espírito Santo Basin, which extends over ca. 630 km2. Together, these zones have the capacity to store > 6.1 Gt CO2 providing Brazil with suitable capacity for reducing its CO2 emissions over the short- to medium-term.
- Article
(7210 KB) - Full-text XML
-
Supplement
(942 KB) - BibTeX
- EndNote
Global warming is poised to be humanity's biggest challenge in the 21st century, requiring a significant reduction in anthropogenic CO2 emissions to mitigate climbing global temperatures. Year after year, the Intergovernmental Panel on Climate Change, IPCC, predicts scenarios of slow to rapid increases in the global average temperature, driven primarily by increasing concentrations of greenhouse gasses (e.g. IPCC, 2014, 2023). Several governments worldwide, including Brazil, have committed to achieving net-zero CO2 emissions by 2050, which is virtually impossible without carbon capture and storage deployment (Bains et al., 2020; IPCC, 2023). Carbon Capture and Storage (CCS) is a set of technological solutions or processes that capture and sequester CO2, thereby reducing total CO2 emissions into the atmosphere (e.g., Gibbins and Chalmers, 2008; Oelkers et al., 2008). Captured CO2 is compressed and transported to sites where it is injected into deep geological formations. Depleted oil and gas reservoirs and saline aquifers are the primary options for permanent CO2 storage underground.
The potential for CO2 storage in saline aquifers has proven successful in several sites, with notable examples including Sleipner and Snøhvit in Norway, In Salah in Algeria, and Ketzin in Germany (e.g., Prevedel et al., 2008; Wildenborg et al., 2013; Chadwick et al., 2014). The Sleipner project has been monitored closely for over two decades and stands as a successful case wherein 16 Mt of CO2 has been securely contained in a saline aquifer 800 m below the seafloor (Furre et al., 2020). When compared to depleted oil and gas fields, CCS in saline aquifers not related to depleted oil/gas reservoirs offers significant advantages. These saline aquifers are generally more widespread and accessible to capture sites and are often located at shallower depths, which together reduce transportation and injection costs. Saline aquifers typically also have higher storage capacity and fewer well penetrations than depleted oil and gas reservoirs, the latter of which lowers the risk of CO2 leakage out of the reservoir. In contrast, injection in depleted oil and gas fields may cause unexpected high-pressure buildup if a significant amount of gas remains in the field (Chadwick et al., 2008; Raza et al., 2018).
Brazil is ranked 7th in global CO2 emissions and 1st in South America, having emitted over 1500 Mt CO2 in 2021 (with 32.2 % of this attributed to fossil fuels; Climate Watch, 2024), and yet the country is in the early stages of developing and applying CCS. Presently, CO2 injection is occurring only in the Santos Basin and for enhanced oil recovery (Ciotta et al., 2022). There is only limited exploration of CO2 injection elsewhere in Brazil. Brazil presents great potential for geological CO2 storage given that there are 31 sedimentary basins that extend over approximately 6.4 million km2 (75 % of which is situated onshore; Fig. 1).
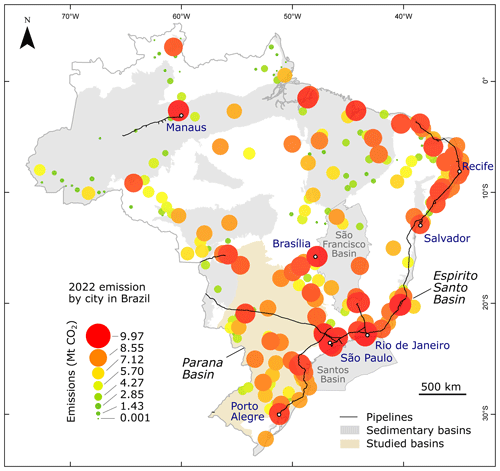
Figure 1Map of Brazil with the extent of onshore sedimentary basins, CO2 emissions by city in 2022 (SEEG, 2023), and existing pipelines that can be used for CO2 transportation. The only existing CO2 injection project in Brazil is in the Santos Basin and is done for enhanced oil recovery (Ciotta et al., 2022).
Herein, we use publicly available data (geological maps, roads, gas/oil pipelines and well logs) to assess the logistics and feasibility of utilizing saline aquifer in two onshore intracratonic basins of southern Brazil as CO2 sinks. We scrutinize these sedimentary basins and sedimentary strata therein for their CCS potential based on depth, thickness and lithological composition, and their proximity to existing pipelines. Southern Brazil is focused on because it is the epicenter of stationary (i.e., from industrial processes and energy processing) CO2 emissions in the country (Fig. 1; SEEG, 2023), and our main objective is to show the potential for CCS in Brazil as the country works towards its goal of net-zero CO2 emissions by 2050.
CO2 emissions in southern Brazil surpass those of the rest of the country by more than two times (280 503 kt CO2 vs. 138 293 kt CO2; SEEG, 2023). Within southern Brazil, three tectonically stable, onshore intracratonic basins contain saline aquifers: São Francisco, Paraná and Espírito Santo basins (Fig. 1). The São Francisco Basin is not evaluated herein because there are no existing gas or oil pipelines to transport CO2. Consequently, we focus on the two most promising candidates for CO2 injection: Paraná Basin and the onshore portion of Espírito Santo Basin (Fig. 2).
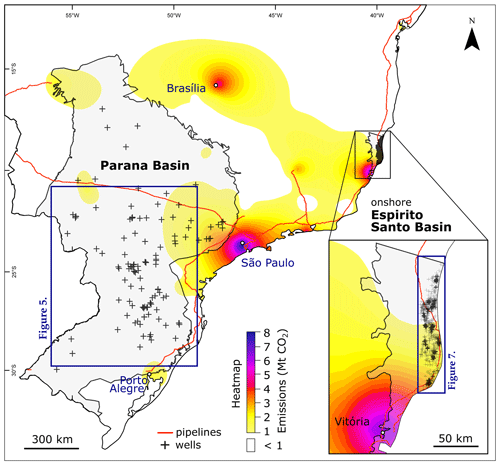
Figure 2Map of southern Brazil showing the studied basins, available wells in the Brazilian National Agency for Petroleum, Natural Gas and Biofuels (ANP) database, pipelines, and CO2 emissions from stationary sources (SEEG, 2023).
The Paraná Basin is the most studied Brazilian intracratonic basin for CCS due to its extensive area, thick sedimentary succession, and strategic geographical location (i.e., proximity to cities with the largest concentrations of stationary CO2 emitting sources; Figs. 1 and 2; e.g. Ketzer et al., 2009; Rockett et al., 2011; Lima et al., 2011; Machado et al., 2013; Orita and Da Cruz, 2022; Oliveira et al., 2023; Abraham-A et al., 2024; Weber et al., 2024). In this basin, we analyze the Permian Rio Bonito Formation (Fm) because previous studies have confirmed this formation contains saline aquifers with over 10 000 ppm of total dissolved solids (Ketzer et al., 2009; Lima et al., 2011; Moreira et al., 2016; Machado and Freitas, 2005). This concentration is significantly above the 5000 ppm limit for drinking water set by the Brazilian National Environment Council.
In contrast to the Paraná Basin, there are no previous studies on CCS in the Espírito Santo Basin. In this basin, we chose to analyze the Early Cretaceous Mucuri Member based on our verification of formation testing analyses within well reports from the Brazilian National Agency for Petroleum, Natural Gas and Biofuels (ANP; see Sect. 3, “Dataset and Methodology”), which confirmed this unit is saturated with saline water.
2.1 Paraná Basin
The Paraná Basin is a Phanerozoic intracratonic basin that originated from a Neo-Ordovician rift (Zalán et al., 1990). The basin is elongated in a general N–S direction, and extends over 1 400 000 km2 across Brazil, Argentina, Uruguay, and Paraguay (Milani et al., 2007). Two prominent lineament trends are evident in the Brazilian portion of the basin: NE–SW oriented lineaments and NW–SE oriented ones (Zalán et al., 1990; Scherer et al., 2023) (Fig. 3a). The NE–SW trend corresponds to structural reactivation during the Paleozoic due to renewed compressional stress induced by the accretion of terrains along the western margin of Gondwana (Holz et al., 2006). The NW–SE trend corresponds to strike-slip zones along which intracontinental movements accommodated the regional stress field associated with the rifting of the South Atlantic (Zalán et al., 1990). The basin infill reaches 7000 m and comprises six supersequences, which represent phases of subsidence and sedimentation from the Ordovician through to the Cretaceous (Milani et al., 1998, 2007). Our work focuses on the third supersequence, Gondwana I (Carboniferous to Eotriassic) (Fig. 3b). It constitutes the largest sedimentary strata volume in the Paraná Basin with a thickness of up to 2500 m (Milani, 1997). The basal units (Itararé Group and Aquidauana Fm) comprise glaciogenic deposits related to deglaciation in the Mississippian to Pennsylvanian. Deglaciation caused a rise in relative sea level, historically known as the “Permian Transgression” (Lavina and Lopes, 1987). The transgressive trend is recorded by sedimentary strata of the Guatá Group (Permian; Fig. 3c), including the Rio Bonito and Palermo formations (Fig. 3b).
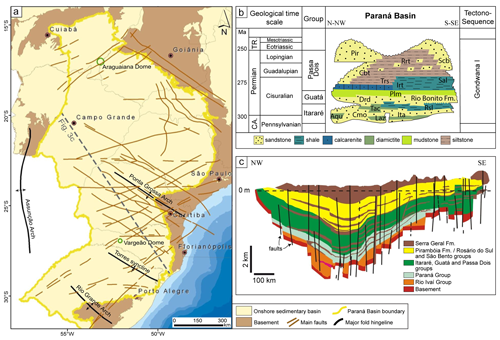
Figure 3(a) Map of structures in the Paraná Basin (modified from Zalán et al., 1990). (b) Stratigraphic chart of the Paraná Basin (modified from Milani and Zalán, 1998). Acronyms include: Aquidauana Fm (Aqu), Campo Mourão Fm (Cmo), Carboniferous (CA), Corumbataí Fm (Cbt), Dourados Fm (Drd), Irati Fm (Irt), Itararé Group (Itr), Lagoa Azul Fm (Laz), Parlermo Fm (Plm), Pirambóia Fm (Pir), Rio do Rasto Fm (Rrt), Rio do Sul Member (Rsl), Sanga do Cabral Fm (Scb), Serra Alta Fm (Sal), Taciba Fm (Tac), Teresina Fm (Trs), Triassic (TR.). (c) NW-SE schematic geological cross-section of the Gondwana I Supersequence of the Paraná Basin (modified from Milani et al., 2007); its location is highlighted with a gray dashed line in panel (a).
The Rio Bonito Fm is present in the central-southern Paraná Basin, including areas of the Brazilian states of (from north to south) São Paulo, Paraná, Santa Catarina and Rio Grande do Sul (Oliveira et al., 2023). The formation consists of paralic (deltaic, estuarine and shallow marine) fine- to coarse-grained sandstone, mudstone and coal (Milani et al., 2007). Base-level oscillations during deposition of the Rio Bonito Fm produced a notable sedimentary cyclicity of predominantly sandy and muddy intervals (Milani et al., 2007). The overlying Palermo Fm comprises sandy siltstone, siltstone and shale, locally interbedded with lenticular sandstone layers (Milani, 1997). All sedimentary sequences of the Paraná Basin, including the Rio Bonito and Palermo formations, were later intruded by a network of dikes and sills during the Early Cretaceous Serra Geral volcanism (Milani et al., 2007).
The Rio Bonito Formation has been an important target for mining and petroleum exploration since the early 20th century. Coal seams in the Rio Bonito Fm were recognized early on as Brazil's most valuable coal deposits (White, 1908). Coal production has been ongoing since the early 20th century, although production is currently declining. In the 1980s, the Rio Bonito Formation became a focus for petroleum exploration (Paulipetro, 1982; Zalán et al., 1990), with companies exploring for trapped oil released from the Irati (Permian) shale source rock. This exploration led to the discovery of the relatively small Barra Bonita Field in 2016, which remains undeveloped to this day (ANP, 2024).
Comparative Analysis of CO2 Storage Potential in the Rio Bonito Fm
Previous studies have targeted the Rio Bonito Formation for CO2 storage (e.g., Oliveira et al., 2023; Abraham-A et al., 2024; Ketzer et al., 2009; Lima et al., 2011). Abraham-A et al. (2024) analyzed the Rio Bonito Fm in the São Paulo State, focusing on physical properties such as porosity, permeability, and sandstone thickness. Abraham-A et al. (2024) findings indicate that porosity and permeability values are satisfactory for CO2 injection, with porosity ranging mostly between 10 % and 30 % and effective permeability reaching 10 000 mD. Sandstone layers in the region vary in thickness from 7–24 m and are often interbedded with thin siltstone layers. According to Abraham-A et al. (2024), these thin siltstone units are unlikely to significantly impact CO2 injection and circulation, and a hybrid reservoir involving sandstone-siltstone-sandstone sequences is feasible in areas with thinner sandstone layers.
Oliveira et al. (2023) conducted a 3D stratigraphic analysis of 125 wells to define formation tops and subdivide the Rio Bonito Fm into three members (Mb): Siderópolis, Triunfo and Paraguaçu (following Holz, 2003). The Siderópolis and Triunfo members comprise mainly sandstone and are considered potential CO2 reservoirs. The Paraguaçu Mb is dominated by shale and siltstone and serves as a caprock. Oliveira et al. (2023) proposed and spatially delimited two systems: Siderópolis Mb (reservoir) – Palermo Fm (caprock); and, Triunfo Mb (reservoir) – Paraguaçu Mb (caprock). However, Oliveira et al. (2023) also indicate that the subdivision of the Rio Bonito Fm into members is imprecise, given: (i) it is based solely on lithology logs; and, (ii) the precise delimitation of each member is challenging due to the spatial continuity between wells.
Ketzer et al. (2009) investigated the potential for CO2 storage in the Rio Bonito Fm using mineral trapping (i.e., via the precipitation of carbonate minerals formed by the reaction of dissolved CO2 with metal cations; Rackley, 2017). The authors performed both experimental and numerical modelling using sandstone with the aim of assessing the mineralogical integrity of the sandstone reservoirs during CO2 injection and storage. The findings of Ketzer et al. (2009) indicate that the sandstones of the Rio Bonito Fm are suitable reservoirs for CO2 storage due to their stable mineralogy, which is primarily composed of monocrystalline quartz grains and, to a lesser extent, potassium feldspar grains. However, the effectiveness of mineral trapping of CO2 is limited due to the low availability of cations necessary for carbonate precipitation. This leads to the conclusion that the most effective trapping mechanisms in saline aquifers of the Rio Bonito Fm are stratigraphic and/or structural.
2.2 Espírito Santo Basin
The Espírito Santo Basin is a passive margin basin that originated during the break-up of the Gondwana Supercontinent and the opening of the South Atlantic Ocean in the Late Jurassic/Early Cretaceous (Szatmari, 2000). The basin extends over approximately 41 500 km2, of which approximately 3000 km2 is located onshore (Fig. 4a). It is bound to the north by the Mucuri Basin along a politically defined boundary, and to the south by the Campos Basin, which is separated from the Espírito Santo Basin by the Vitoria High (Vieira, 1998).
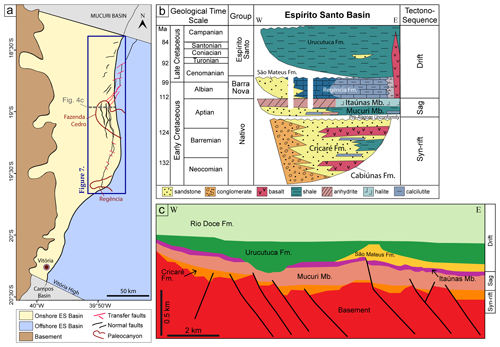
Figure 4(a) Simplified structural map of the onshore Espírito Santo Basin (with data from Vieira, 1998, and Amarante et al., 2020). (b) Stratigraphic chart of the syn-rift, sag and post-rift/drift tectono-sequences of the Espírito Santo Basin (modified from França et al., 2007). (c) Schematic geological section of the Paraná Basin (modified from Neves et al., 2018); its location is highlighted with a gray dashed line in panel (a).
The sedimentary infill of the Espírito Santo Basin is divided into syn-rift, sag and post-rift/drift stages (Mohriak, 2003). The syn-rift tectono-sequence (Neocomian–Early Aptian; Fig. 4b) is associated with the formation of N–S to NE–SW-trending normal faults that bound horsts and grabens (Fig. 4a, c). Sedimentation in this stage comprises alluvial and fluvial-lacustrine sediments intercalated with volcanic and volcaniclastic rocks of the Cricaré Fm (Vieira, 1998; França et al., 2007).
The early post-rift (i.e., sag) stage initiated in the Aptian (Fig. 4b) and marked the cessation of most basement-involved fault activity and the beginning of thermal subsidence (Karner et al., 2003). Sedimentary strata deposited during this stage include the Mariricu Fm, which is subdivided into the Mucuri Member (the focus of this study) and the Itaúnas Member (Asmus et al., 1971; Mohriak, 2003). The Mairircu Fm unconformably overlies either crystalline basement over structural highs (i.e. horsts) or syn-rift deposits of Cricaré Fm in structural lows (i.e. grabens; Fig. 4b).
The Mucuri Member (Aptian) is composed of arkosic to lithic sandstone and conglomerate deposited in coastal environments, intercalated with lacustrine shale, siltstone and evaporites (Fig. 4b; França et al., 2007). The thickness of the Mucuri Mb reaches 2000 m towards the basin depocenter but is highly variable due to deposition over remnant rift-stage topography (Fig. 4c; França et al., 2007; Amarante et al., 2020). The overlying Itaúnas Mb consists of thick evaporitic successions composed of dominantly halite and anhydride that were deposited in response to episodic marine water influx into a restricted basin (Vieria, 1998; Davison et al., 2012). The thickness of the Itaúnas Mb varies from 50 m in the onshore extent of the Espírito Santo Basin to 2000 m in the offshore extent (Karner and Gamboa, 2007). Paleocanyons (Fazenda Cedro and Regência canyons) that formed during an Upper Cretaceous regression completely eroded through the Itaúnas Mb, resulting in the Mucuri Mb being unconformably overlain by turbiditic sandstone and shale (Fig. 4a, c; Mohriak, 2003; Amarante et al., 2020).
The Mucuri Mb has been the focus of significant petroleum discoveries, resulting in production from 41 small onshore oil fields between 1969 and 2022 (ANP, 2024). Most of these fields are now nearing depletion or are fully depleted and are operated by independent small Brazilian companies. Due to the extensive exploration effort in the area, the Espírito Santo Basin is well-characterized with over 1500 drilled wells and a pipeline system that runs north-south across its onshore portion (EPE, 2024).
We use publicly available infrastructure data (roads and gas/oil pipelines) from MapBiomas Brasil (https://brasil.mapbiomas.org/dados-de-infraestrutura/, last access: 20 March 2024) and publicly available well-logs, well reports and sedimentary basin maps from the Brazilian National Agency for Petroleum, Natural Gas and Biofuels (ANP) (https://reate.cprm.gov.br/anp/TERRESTRE, last access: 20 March 2024) to generate maps, and analyze lithology, composition and porosity values. Specifically, we utilized data from 79 wells in the Espírito Santo Basin and 93 wells in the Paraná Basin that have complete or partial lithological and compositional data for the Mucuri Member (Espírito Santo Basin) and Rio Bonito Fm (Paraná Basin).
To assess the suitability of a saline aquifer within a geological formation for long-term carbon storage, a comprehensive geological evaluation is necessary to ensure that target zones meet critical parameters. Adopting the classification of phases of CO2 storage projects outlined by Goodman et al. (2011), the evaluation presented herein corresponds to the site screening phase, where selected areas are identified based on regional and local geological data.
The Mucuri Mb and Rio Bonito Fm are the focus on herein based on a bibliographic review and a preliminary analysis of formation testing reports in the well data provided by ANP, both of which confirm the presence of saline water. Our assessment considers depth, reservoir thickness, and caprock thickness. A minimum depth of 800 m is used as this is the depth at which CO2 reaches a supercritical state under typical geothermal gradients for intracratonic basins (25 °C km−1) and under normal hydrostatic pressure gradients (Holloway and Savage, 1993; Aminu et al., 2017). A maximum depth of 2500 m is used to capture all reservoir units with adequate porosity and permeability (Chadwick et al., 2008), and because injection costs increase substantially with depth (Kolenović and Saftic, 2014). It is important to note that we use the top surface of the target units to determine depth cutoffs (between 800 and 2500 m). A reservoir thickness greater than 20 m is suggested by the International Energy Agency (IEA GHG, 2009) for CO2 sequestration. We use a minimum of 20 m but follow the approach of Chadwick et al. (2008) and consider cumulative sandstone bed thicknesses of over 20 m instead of individual bed thicknesses. Finally, we identify areas where reservoir rocks are overlain by caprocks (seals) that have a minimum thickness of 10 m (IEA GHG, 2009). Contour maps were generated using ArcGIS software; well-log analysis and well correlation were done using Petrel (Schlumberger reservoir modelling software).
We also conducted a preliminary analysis of porosity variation using publicly available porosity data from well reports provided by ANP (Tables S1 and S2 in the Supplement). The data varies between wells: some reports include porosity based on individual petrophysical logs, others provide the average porosity based on multiple petrophysical logs, and some include porosity data from lab analysis of core samples. The latter is preferred due to its higher precision. In saline aquifers, 20 % porosity is ideal, although values above 10 % can be considered with caution (Chadwick et al., 2008). Lastly, we estimate the average CO2 storage capacity using the method by van der Meer and Yavuz (2009), multiplying the reservoir area, the sandstone thickness, the efficiency factor (constant: 0.02, i.e. 2 % of the pore volume is effectively available for CO2 storage), the porosity and the CO2 density at depth (in average 600 kg m−3).
4.1 Paraná Basin
The Rio Bonito Fm is found in wells across the central-southern Paraná Basin (Fig. 5). Its top surface ranges from outcrop exposures to over 3900 m depth, deepening towards the west (Fig. 5a). The ideal depth for CO2 injection, between 800 and 2500 m, occurs along a N–S trending belt and in the southern area (Fig. 5a). The formation's thickness varies from 25–350 m and is greatest in four areas (labelled 1 to 4 in Fig. 5b). The cumulative thickness of sandstone layers within the Rio Bonito Fm ranges from 13–150 m (Fig. 5c) and is greater in four areas that are aligned along a NW–SE trend (labelled 1 to 4 in Fig. 5c), corresponding to three of the areas where the formation is thickest (2 to 4 on Fig. 5b). In most areas of the basin, the caprock comprises shale and siltstone of the Palermo Fm (Figs. 3b and 5d – green and brown dots). Previous studies (e.g., Maskell et al., 2018; Jayasekara et al., 2020) have shown that siltstone is an effective seal for CO2 entrapment, and hence the caprock is deemed to be suitable where the combined thickness of shale and siltstone beds exceeds 10 m (Fig. 5d). Locally, the thickness of the effective seal is zero because the lithology directly above Rio Bonito Fm is a sandstone (Fig. 5d – yellow dots). The thickness of effective sealing lithologies (shale and siltstone) ranges between 2 and 214 m, but is most commonly between 10 and 82 m.
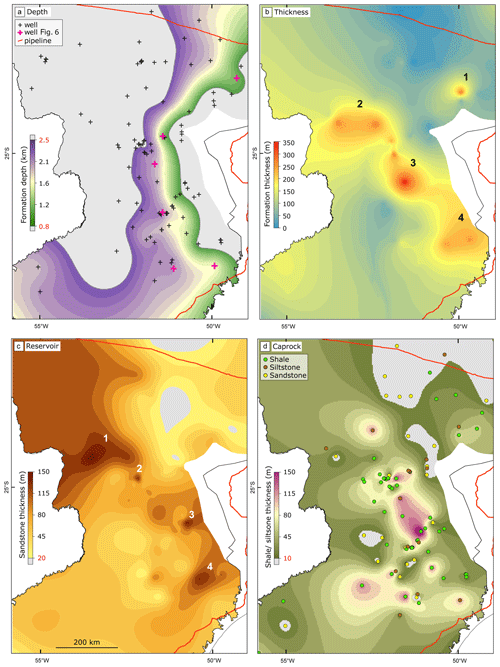
Figure 5Maps of the Rio Bonito Fm in the central Paraná Basin (see Fig. 2 for location). (a) Surface map of the top of the Rio Bonito Fm Regions with values outside the ideal depth for CO2 storage (between 800 and 2500 m) are coloured in grey. (b) Total thickness of the Rio Bonito Fm. (c) Cumulative thickness of sandstone layers within the Rio Bonito Fm; regions with thicknesses less than 20 m are coloured in grey. (d) Caprock thickness (i.e., the thickness of the lithology immediately above the Rio Bonito Fm). Regions with thicknesses less than 10 m and regions where an effective seal is absent are coloured in grey. Some areas are numbered to facilitate linkages to descriptions in the text.
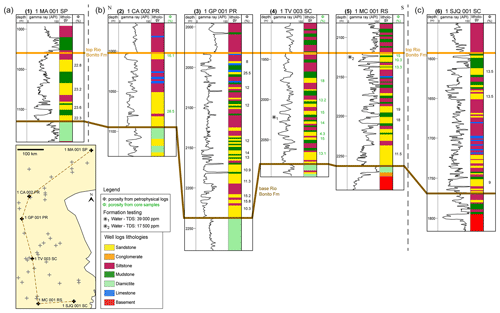
Figure 6Stratigraphic cross-section through part of the Paraná Basin with gamma-ray curves, lithology, porosity values determined from cores (in green) or petrophysical logs (in black), and the top and base of the Rio Bonito Fm. The top of Rio Bonito Fm is used as the datum, and the depth values represent true vertical depth. (a) Northeastern extent of basin represented by Well 1 MA 001 SP. (b) Central Paraná Basin represented by wells 1 CA 002 PR, 1 GP 001 PR, 1 TV 003 SC, and 1 MC 001 RS. (c) Southwestern extent of the basin represented by Well 1 SJQ 001 SC. See the map in the lower left and Figs. 5a and 9 for well locations. The wells are numbered to facilitate linkages to descriptions in the text.
Figure 6 shows six wells considered representative of the Rio Bonito Fm in the northeastern, central and southern parts of the Paraná Basin. In the northeastern well (Fig. 6a – well 1), the top Rio Bonito Fm is at 1030 m and the interval is 85 m thick. The cumulative sandstone thickness is 43 m and the thickness of individual sandstone layers increases towards the top of the unit, reaching 15 m (Fig. 6a). The porosity of sandstone layers varies between 22.3 % and 23.6 % (Fig. 6a). In the central Paraná Basin (Fig. 6b), the top Rio Bonito Fm ranges in depth between 1002 and 2118 m. The formation thickness varies from 94–210 m, and the cumulative sandstone thickness ranges from 41–80 m (wells 3 and 4, respectively). The thickness of individual sandstone layers shows an overall increase towards the base of the unit, with individual intervals reaching a gross sandstone thickness of 45 m and a net thickness of 27 m in the northernmost well, and a net thickness of 25 m in the southernmost well (Fig. 6b – wells 2 and 5). The porosity of sandstone layers varies from 4.3 %–28.5 % and is greater overall in the northernmost well (Fig. 6b – well 2) where values average 21.8 %. In the three southernmost wells in the central Paraná Basin, the porosity averages between 13.2 % and 14 % (Fig. 6b). Formation testing in the two southern wells of the central Paraná Basin shows intervals saturated with saline water, with total dissolved solids (TDS) of 17 500 and 39 000 ppm (Fig. 6b). In the well representative of the southwestern Paraná Basin (Fig. 6c – well 6), the top Rio Bonito Fm is 1593 m deep and has a thickness of 176 m. The cumulative sandstone thickness is 53 m, and the thickness of individual sandstone layers ranges from 2–9 m (Fig. 6c). The porosity of sandstone layers varies between 9 % and 13.5 % (Fig. 6c). Across all six wells, siltstones interbedded with shales and rarely limestones overlay the Rio Bonito Fm (Fig. 6).
4.2 Espírito Santo Basin
The Mucuri Mb is present throughout nearly all of the onshore extent of the Espírito Santo Basin. Its top surface ranges in depth between 500 and 2650 m and deepens towards the SE. The ideal depth for CO2 injection, between 800 and 2500 m, occurs across nearly the entire basin (Fig. 7a). The thickness of Mucuri Mb ranges from 3–400 m, with thicker sequences located in two areas in the central portion of the Espírito Santo Basin (labelled 1 and 2 in Fig. 7b), and thinner intervals to the north and in the vicinities of the paleocanyons (Figs. 4a and 7b). The cumulative thickness of sandstone layers within the Mucuri Mb ranges from 0–320 m and is greater in the two central regions where the Mucuri Mb is the thickest (areas 1 and 2 in Fig. 7c). In most areas of the basin, the caprock comprises evaporites of the Itaúnas Mb which range in thickness from 5–253 m, but are mainly 10–85 m thick (Fig. 7d – pink dots). In three wells, the lithology directly above the Mucuri Mb is shale, with thickness values of 781, 12 and 25 m from north to south, respectively (Fig. 7d – green dots). Locally, the caprock is not an effective seal as the lithology directly above the target unit is a sandstone (Fig. 7d – yellow dots).
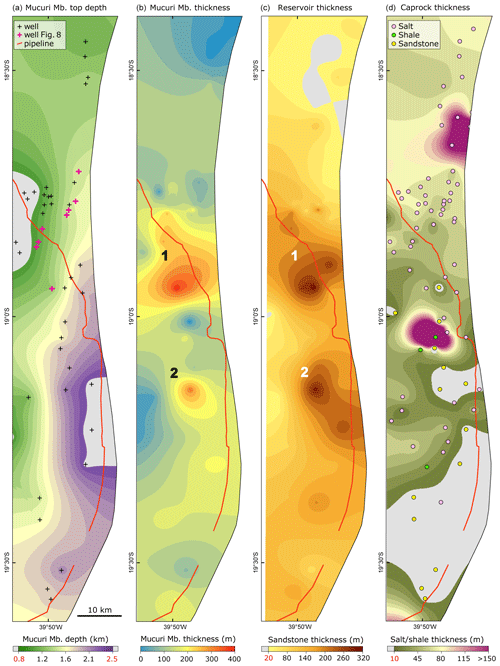
Figure 7Maps of the onshore portion of Espírito Santo Basin. (a) Surface map of the top of the Mucuri Member (Mb); regions with values outside the ideal depth for CO2 storage (between 800 and 2500 m) are coloured in grey. (b) Total thickness of the Mucuri Mb. (c) Cumulative thickness of sandstone layers within the Mucuri Mb; regions with thicknesses less than 20 m are coloured in grey. (d) Caprock thickness (i.e. the thickness of the lithology immediately above the Mucuri Mb). Regions with caprock thicknesses less than 10 m and regions where an effective seal is absent are coloured in grey. Some areas are numbered to facilitate linkages to descriptions in the text.
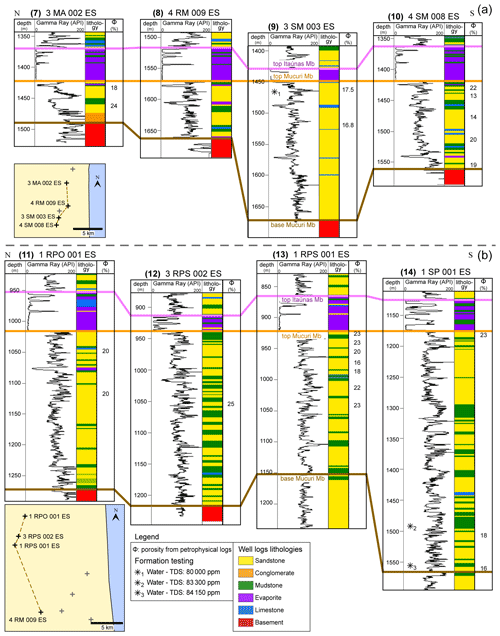
Figure 8Stratigraphic cross-sections through parts of the onshore part of the Espírito Santo Basin, with gamma-ray curves, lithology, porosity values determined from petrophysical logs, and the top and base limits of the target unit (Mucuri Member (Mb)) and caprock unit (Itaúnas Mb). (a) Correlation of wells: 3 MA 002 ES, 4 RM 009 ES, 3 SM 003 ES, and 4 SM 008 ES. (b) Correlation of wells 1 RPO 001 ES, 3 RPS 002 ES, 1 RPS 001 ES and 1 SP 001 ES. Both correlations use the top Mucuri Member as datum, and the depth values represent the true vertical depth. See Figs. 7a and 9 for location of the cross sections with the Espírito Santo Basin. The wells are numbered to facilitate linkages to descriptions in the text.
Figure 8 displays two stratigraphic cross-sections across the north-central region of the onshore Espírito Santo Basin. In the northern section (Fig. 8a), the top of the Mucuri Mb ranges in depth from 1420–1570 m. Thickness values are between 67 and 222 m, with lower values in the two northernmost wells. Sandstone layers are interbedded with shale and limestone and less commonly with conglomerate and thin layers of evaporites. The cumulative sandstone thickness ranges from 35–211 m (wells 7 and 9, respectively). The thickest individual sandstone layers are found in well 9, reaching 72 m of net sandstone thickness (222 m of gross sandstone thickness). The average porosity in wells in the northern onshore Espírito Santo Basin varies between 13 % and 24 %. Formation testing in well 9 show an interval saturated with saline water, with total dissolved solids (TDS) of 80 000 ppm (Fig. 8a). In the central part of the onshore Espírito Santo Basin (Fig. 8b), the Mucuri Mb is at shallower depths, with the top of the interval ranging from 922–1175 m deep. Thicknesses of the Mucuri Mb in this region exceed those in the northern section and vary from 227–393 m. Sandstone layers are interbedded with shale and less commonly with limestone and evaporites. The cumulative sandstone thickness ranges from 170–267 m (wells 13 and 14, respectively). The thickness of individual sandstone layers varies significantly, and is greatest in wells 11 (84 m gross thickness, 65 m net thickness) and 14 (90 m gross thickness, 44 m net thickness). The average porosity in the central part of the onshore Espírito Santo Basin varies between 16 % and 25 %. Formation testing in well 14 shows two intervals saturated with saline water, with TDS of 83 300 and 84 150 ppm (Fig. 8b). Across all eight wells, evaporites of the Itaúnas Mb overlie the Mucuri Mb (Fig. 8).
It is estimated that CCS can be applied to reduce up to 190 million tonnes of CO2 annually in Brazil (CCS-Brasil, 2023), and the site screening we present herein (sensu Goodman et al., 2011), identifies areas that are best suited for CO2 storage in saline aquifers within the Paraná Basin and onshore extent of the Espírito Santo Basin. Formation testing data from ANP reveals that both the Rio Bonito Fm (Paraná Basin) and Mucuri Mb (onshore portion of the Espírito Santo Basin) are saturated with saline water (Tables S1 and S2 in the Supplement), with a mean total dissolved solids (TDS) above 5000 ppm, which is the maximum TDS limit in drinking water, as determined by the Brazilian National Environment Council.
5.1 Rio Bonito Formation, Paraná Basin
The Rio Bonito Fm contains areas that have the potential for CO2 injection in the north, central and southern portions of the Paraná Basin (Fig. 9a). These areas are identified by overlaying the depth, sandstone thickness and caprock thickness maps (Fig. 5), and areas where all 3 attributes are favorable (depth: 800–2500 m; cumulative sandstone thickness > 20 m; caprock > 10 m) are shown in green. In the areas defined as potential for CO2 injection, the sandstone porosity of Rio Bonito Fm has a mean value of 14.8 %, and formation water has a mean salinity of 36 000 ppm of TDS (Table S1 in Supplement). We consider two zones to be optimal for CO2 injection due to their proximity to pipelines (within 200 km; labeled 1 and 2 on Fig. 9a). Zone 1 was previously studied by Abraham-A et al. (2024) and is discussed below.
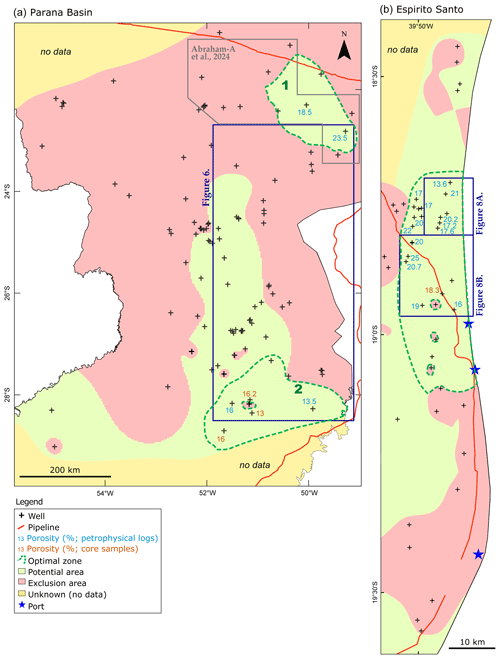
Figure 9Maps of the (a) Paraná Basin, and (b) Espírito Santo Basin showing zones that have potential for CO2 injection (in green) and excluded zones (in red). These zones are defined using parameters outlined by IEA GHG (2009) and Chadwick et al. (2008). Areas with no well data are in yellow. The blue rectangles indicate the location of the maps shown in Figs. 6 and 8; the gray polygon includes the wells in Abraham-A et al. (2024). Two areas are numbered to facilitate indications in the text.
Zone 1 constitutes the northeastern portion of the study area and extends over 27 700 km2 (Fig. 9a). In this zone, the Rio Bonito Fm varies in depth between 800 and 2300 m. The cumulative sandstone thickness ranges from 20–50 m, averaging 35 m. The caprock is 15–50 m thick (Fig. 5). The average porosity in zone 1 is 21 % as determined from petrophysical logs (Fig. 9a). Figure 6 shows one well representative of this zone (well 1 in Fig. 6a) which has a cumulative sandstone thickness of 43 m, and the thickness of individual sandstone layers reaches 15 m. The mean porosity of sandstone layers is 23.5 % (Fig. 6a). Considering the average cumulative sandstone thickness of 35 m and the average porosity of 21 %, we estimate that zone 1 can have a storage capacity of about 2.4 Gt CO2.
As aforementioned (in Sect. 2.1), the area with potential for CO2 injection in the northeast, (zone 1 in Fig. 9a) was previously studied by Abraham-A et al. (2024). The authors concluded that Rio Bonito Fm has the potential for CO2 storage across their entire study area (delineated in gray in Fig. 9a), wherein porosity ranges mostly between 10 % and 30 %. However, it is important to note that the authors did not account for reservoir depth (ideally between 800 and 2500 m), lithology, and caprock effectiveness as a seal. Additionally, Abraham-A et al. (2024) did not present porosity values for individual wells, and it is unlikely that wells where the unit is greater than 2500 m deep will have porosity as high as 30 % (Chadwick et al., 2008). We refine the findings of Abraham-A et al. (2024) to exclude shallow and deep reservoirs and to account for areas with caprock greater than 10 m. Based on this, we reduce the extent of zone 1 that is suitable for CO2 injection (Fig. 9a).
Zone 2 is located in the southern Paraná Basin and extends over 38 100 km2 (Fig. 9a). In this zone, the Rio Bonito Fm varies in depth between 800 and 2200 m. The cumulative sandstone thickness ranges from 20–120 m with an average thickness of 70 m. The caprock comprises shale and siltstone that range from 15–120 m thick (Fig. 5). The average porosity in this zone is 15 %, as determined from core samples and petrophysical logs (Fig. 9a). Figure 6 shows two wells in this zone: numbers 5 and 6. Well 5 (Fig. 6b) shows a cumulative sandstone thickness of 72 m, and the net thickness of individual layers increases to the base of the well, reaching 25 m. The mean porosity value is 13 %. The well in the southeast (well 6 in Fig. 6c), has a cumulative sandstone thickness of 53 m, and the sandstone layers in this well are thinner, ranging from 2–9 m. The mean porosity value is 13.5 %. Considering the average cumulative sandstone thickness of 70 m and an average porosity of 15 %, we estimate that zone 2 can store about 4.8 Gt CO2.
It is important to note that the estimated CO2 storage capacities presented in our study do not account for the lateral connectivity of the sandstone bodies. As discussed in Sect. 2.1, the Rio Bonito Formation is composed of paralic depositional systems, including deltaic, estuarine and shallow marine systems (Milani et al., 2007). Several studies on the Rio Bonito Formation (Maahs et al., 2024, 2023, 2019; Holz et al., 2006; Holz, 2003; Holz and Carlucci, 2000; Kuchle and Holz, 2002; Holz, 1998) suggest that these paralic environments typically exhibit low lateral continuity in sandstone facies due to the nature of depositional settings such as shoreface (upper to middle), delta fronts, tidal sand bars, fluvial channels, and barrier deposits. These sandy architectural elements are usually around 100–300 m wide (as modeled by Maahs et al., 2024). However, despite this heterogeneity and complexity, there is evidence of moderate to good lateral connectivity between these sand bodies, largely due to the high sandstone content (30 %–60 %, Fig. 5b, c), high amalgamation levels (Maahs et al., 2024), and localized diagenetic cementation (Ketzer et al., 2003). This enhanced connectivity could facilitate the formation of continuous flow units extending over distances of up to 10 km. The amalgamated sand bodies within this context display a “jigsaw puzzle” heterogeneity and flow pattern, as described by Galloway and Hobday (1996).
5.2 Mucuri Member, Espírito Santo Basin
The second saline aquifer analyzed in this study is the Mucuri Mb of the onshore Espírito Santo Basin, and based on our site-screening criteria, the Mucuri Mb has the potential for CO2 injection in most of the basin (green areas in Fig. 9b). In the areas defined as having potential for CO2 injection, sandstone porosity of Mucuri Mb averages 18.4 % and formation water has a mean salinity of 43 610 ppm of total dissolved solids (Table S2 in the Supplement) We consider the central-north area of the basin to be the optimal zone for CO2 injection due to its proximity to an existing pipeline (0–20 km) and density of wells and well-based data (e.g., well logs; Fig. 9b). The optimal zone extends over 630 km2 , and within that zone: the Mucuri Mb varies in depth between 800 and 2300 m; the cumulative sandstone thickness ranges from 20–320 m (averages 170 m); the average porosity is 19.2 %; and, the caprock, mainly evaporites, ranges from 15–85 m thick (Fig. 7). Figure 8 displays eight wells in this zone. The wells show a cumulative sandstone thickness between 35 and 267 m, with individual layers reaching 72 m of net thickness or up to 222 m of gross thickness. The average porosity between all wells is 19.6 %. Considering the average cumulative sandstone thickness of 170 m and an average porosity of 19.2 %, we estimate that the onshore Espírito Santo Basin has a storage capacity of about 0.25 Gt CO2.
Similar to the Rio Bonito Formation in the Paraná Basin, the Mucuri Member is a highly complex amalgamated system, also associated with paralic environments (Althaus et al., 2020; Pantopoulos et al., 2021; Vieira, 1998; Vieira et al., 1994). The depositional interaction of ephemeral fluvial streams near the basement, along with discrete deltaic protrusions reworked by wave-dominated shoreface and offshore lacustrine processes, results in a high sandstone content, ranging from 50 %–70 % (Fig. 7b, c). The fluvial and wave-dominated conditions create small architectural elements, typically only a few hundred meters wide, but with a very high degree of amalgamation and minimal mudstone interlayers (Pantopoulos et al., 2021). While diagenetic cementation, which can act as flow barriers, is present, it is scattered and discontinuous (Carvalho et al., 2014), exerting only a minor impact on flow effectiveness. As a result, like in the Rio Bonito Fm., the high connectivity between these sand bodies may support continuous flow units over distances exceeding 10 km in a “jigsaw puzzle” heterogeneity and flow dynamics (as described by Galloway and Hobday, 1996).
In contrast to the Paraná Basin, there are no previous studies on CCS in the Espírito Santo Basin, and research on the petrophysical properties of the Mucuri Mb is very limited. Carvalho et al. (2014) analyzed the porosity of the Mucuri Mb in the onshore Espírito Santo Basin, and indicated that intergranular porosity reaches 26.7 % and secondary intragranular porosity reaches 17.3 %, these are satisfactory values for CO2 storage (Chadwich et al., 2008).
We use publicly available data to assess the feasibility of utilizing the saline aquifers within the Rio Bonito Formation of the Paraná Basin and the Mucuri Member of the Espírito Santo Basin as CO2 sinks. The key findings of our study are as follows:
The Rio Bonito Formation is present in the central-southern Paraná Basin. Its top surface ranges from 4 to over 3900 m, increasing towards the west, with thickness varying from 25 to 350 m. The cumulative thickness of sandstone layers ranges from 13 to 150 m. The caprocks are predominantly shales and siltstones, with average thicknesses between 10 and 82 m. However, locally, caprocks can be sandstones, which are not effective sealing rocks. We identified potential areas for CO2 injection in the basin where all 3 attributes are favorable (depth: 800–2500 m; cumulative sandstone thickness > 20 m; caprock > 10 m), and exclusion areas where one or more attributes do not meet the minimum required standards. In the potential areas, the sandstone porosity of Rio Bonito Fm has a mean value of 14.8 %, and formation water has mean a salinity of 36 000 ppm of TDS. Optimal areas for CO2 injection in the Rio Bonito Formation, i.e. potential zones within 200 km of pipelines, are identified in the northeast and southeast portions of the basin. Zone 1, in the northeast, measures over 27 700 km2 , and has an average cumulative sandstone thickness of 35 m and average porosity of 21 %. We estimate this zone has a storage capacity of about 2.4 Gt CO2. Zone 2, in the southeast, measures over 38 100 km2 , has an average cumulative sandstone thickness of 70 m and an average porosity of 15 %. We calculate this zone has a storage capacity of about 3.8 Gt CO2.
The Mucuri Member is present across nearly the entire onshore Espírito Santo Basin. Its top surface ranges from 500 to 2650 m, increasing towards the southeast, with thickness reaching 400 m. The cumulative thickness of sandstone layers reaches up to 320 m. Caprocks are most commonly evaporites, with average thicknesses between 10 and 85 m. Locally, caprocks can be shales (over 12 m thick) or sandstones. Potential areas for CO2 injection show a mean sandstone porosity of Mucuri Mb have a mean porosity 18.4 %, and formation water has mean a salinity of 43 610 ppm of TDS. An optimal zone for CO2 injection in the Mucuri Member is found in the central-north portion of the basin, measuring over 630 km2 and situated within 20 km of pipelines. It has an average cumulative sandstone thickness of 170 m and an average porosity of 19.2 %. We estimate this zone has a storage capacity of about 0.25 Gt CO2.
Our study provides a regional-scale assessment of storage potential in two saline aquifer intervals within two of Brazil's 31 sedimentary basins. The resulting optimal zones defined herein (Fig. 9) encompass more than 65 000 km2 and show significant potential to move to the site selection stage (sensu Goodman et al., 2011). To achieve this, further detailed studies are necessary to refine stratigraphic correlations and improve the characterization of reservoir heterogeneity and quality. These studies should particularly focus on assessing lateral connectivity, defining flow units, and evaluating the effectiveness of permeability to enhance our understanding of the basins' CO2 storage potential. Furthermore, it is necessary to quantify the theoretical storage capacity based on site-specific parameters, determine the probabilities and risks of CO2 leakage through faults and existing well bores, and evaluate operational costs.
All data are publicly available and can be downloaded from the webpages below:
-
Brazil's infrastructure data (roads and gas/oil pipelines): https://brasil.mapbiomas.org/en/dados-de-infraestrutura/ (MapBiomas Brasil, 2024).
-
Dataset of the Paraná Basin (well-logs, well reports and sedimentary basin maps): https://reate.cprm.gov.br/arquivos/index.php/s/z0XoautAuswCSbf (ANP, 2024a).
-
Dataset of the Espírito Santo Basin (well-logs, well reports and sedimentary basin maps): https://reate.cprm.gov.br/arquivos/index.php/s/MSYSOk8HApYAT7w (ANP, 2024b).
The supplement related to this article is available online at: https://doi.org/10.5194/adgeo-65-19-2024-supplement.
FBdA: conceptualization, data curation, formal analysis, investigation, methodology, visualization, writing – original draft preparation. JK: conceptualization, methodology, supervision, visualization, writing – review & editing. CMdSS: resources, supervision, writing – review & editing. SED: methodology, visualization, writing – review & editing. MBH: data curation, methodology.
The contact author has declared that none of the authors has any competing interests.
Publisher's note: Copernicus Publications remains neutral with regard to jurisdictional claims made in the text, published maps, institutional affiliations, or any other geographical representation in this paper. While Copernicus Publications makes every effort to include appropriate place names, the final responsibility lies with the authors.
This article is part of the special issue “European Geosciences Union General Assembly 2024, EGU Division Energy, Resources & Environment (ERE)”. It is a result of the EGU General Assembly 2024, Vienna, Austria, 14–19 April 2024.
The authors gratefully acknowledge the Brazilian National Agency for Petroleum, Natural Gas and Biofuels (ANP) for providing the data. We thank Marc Gil-Ortiz and Johannes Miocic for their constructive insights, and Johannes Miocic, as the topic editor, for his editorial handling.
This paper was edited by Johannes Miocic and reviewed by Marc Gil-Ortiz and Johannes Miocic.
Abraham-A, R. M., San Martín Cañas, S., Miranda, I. F. S., and Tassinari, C. C. G.: Assessment of CO2 storage prospect based on physical properties of Rio Bonito Formation rock units, Energy Geoscience, 5, 100163, https://doi.org/10.1016/j.engeos.2023.100163, 2024.
Althaus, C. E., Scherer, C. M. S., Kuchle, J., Reis, A. D., Ferronatto, J. P. F., De Ros, L. F., and Bardola, T. P.: Wave-dominated lacustrine margin of Aptian pre-salt: Mucuri Member, Espírito Santo Basin, J. S. Am. Earth Sci., 99, 102490, https://doi.org/10.1016/j.jsames.2019.102490, 2020.
Amarante, F. B., Kuchle, J., Iacopini, D., Scherer, C. M. S., Alvarenga, R. S., Ene, P. L., and Schilling, A. B.: Seismic tectono-stratigraphic analysis of the Aptian pre-salt marginal system of Espírito Santo Basin, Brazil, J. S. Am. Earth Sci., 98, 102474, https://doi.org/10.1016/j.jsames.2019.102474, 2020.
Aminu, M. D., Nabavi, S. A., Rochelle, C. A., and Manovic, V.: A review of developments in carbon dioxide storage, Appl. Energ., 208, 1389–1419, 2017.
ANP: Gás Natural e Biocombustíveis, Banco de Dados de Exploração e Produção (BDEP) da Agência Nacional do Petróleo, https://www.gov.br/anp/, last access: 29 June 2024.
Asmus, H. E., Gomes, J. B., and Pereira, A. C. B.: Integração Geológica regional da Bacia do Espírito Santo, in: XXV Congresso Brasileiro de Geologia, São Paulo, Brazil, September 1971, 215–226, 1971.
Bains, P., Baylin-Stern, A., Berghout, N., Budinis, S., Levi, P., Malischek, R., Morgan, T., and Xu, D.: Special Report on Carbon Capture Utilisation and Storage – CCUS in Clean Energy Transitions, International Energy Agency, 120 pp., 2020.
Brazilian National Agency for Petroleum, Natural Gas and Biofuels (ANP): Dataset of the Paraná Basin, Free Access to Public Onshore Technical Data, Brazilian National Agency for Petroleum, Natural Gas and Biofuels [data set], https://reate.cprm.gov.br/arquivos/index.php/s/z0XoautAuswCSbf, last access: 20 March 2024a.
Brazilian National Agency for Petroleum, Natural Gas and Biofuels (ANP): Dataset of the Espírito Santo Basin, Free Access to Public Onshore Technical Data, Brazilian National Agency for Petroleum, Natural Gas and Biofuels [data set], https://reate.cprm.gov.br/arquivos/index.php/s/MSYSOk8HApYAT7w, last access: 20 March 2024b.
Carvalho, A. S. G., Dani, N., De Ros, L. F., and Zambonato, E. E.: The impact of early diagenesis on the reservoir quality of pre-salt (Aptia) sandstones in the Espírito Santo Basin, Eastern Brazil, J. Petrol. Geol., 37, 127–141, 2014.
CCS-Brasil: 1° Relatório Anual de CCS Brasil (1st CCS Brazil annual report), Associação CCS Brasil, 43 pp., 2023.
Chadwick, R. A., Arts, R., Bernstone, C., May, F., Thibeau, S., and Zweigel, P.: Best practice for the storage of CO2 in saline aquifers – observations and guidelines from the SACS and CO2STORE projects, British Geological Survey, Nottingham, UK, 267 pp., ISBN 978-0-85272-610-5, 2008.
Chadwick, R. A., Marchant, B. P., and Williams, G. A.: CO2 storage monitoring: Leakage detection and measurement in subsurface volumes from 3D seismic data at Sleipner, Enrgy. Proced., 63, 4224–4239, 2014.
Ciotta, M., Musarra, R. M. L. M., Tassinari, C. S. G., and San Martín Cañas, S.: CO2 Geological Storage in Santos Basin: Potential and Best Suitable Sites. In: Perspectives to CO2 Geological Storage and Greenhouse Gas Negative Emissions in South-Southeastern Brazil: Paraná and Santos Sedimentary Basins, Blucher, São Paulo, 27–40, ISBN 9786555501346, 2022.
Climate Watch: Total carbon emissions by country, https://www.climatewatchdata.org/, last access: 25 March 2024.
Davison, I., Anderson, L., and Nuttall, P.: Salt deposition, loading and gravity drainage in the Campos and Santos salt basins, Geological Society, London, Special Publications, 363, 159–174, 2012.
Empresa de Pesquisa Energética (EPE): Sistema de Informações Geográficas do Setor Energético Brasileiro, https://www.epe.gov.br/, last access: 29 June 2024.
França, R. L., Del Rey, A. C., Tagliari, C. V., Brandão, J. R., and Fontanelli, P. R.: Bacia do Espírito Santo, Bol. Geociencias Petrobras, 15, 501–509, 2007.
Furre, A., Meneguolo, R., Pinturier, L., and Bakke, K.: Planning deep subsurface CO2 storage monitoring for the Norwegian full-scale CCS project, First Break, 38, 55–60, 2020.
Galloway, W. E. and Hobday, D. K.: Terrigenous clastic depositional systems – applications to fossil fuel and groundwater resources, 2nd edn., Springer, 450 pp., ISBN 978-3642646591, 1996.
Gibbins, J. and Chalmers, H.: Carbon capture and storage, Energ. Policy, 36, 4317–4322, 2008.
Goodman, A., Hakala, A., Bromhal, G., Deel, D., Rodosta, T., Frailey, S., Small, M., Allen, D., Romanov, V., Fazio, J., Huerta, N., McIntyre, D., Kutchko, B., and Guthrie, G.: U.S. DOE methodology for the development of geologic storage potential for carbon dioxide at the national and regional scale, Int. J. Greenh. Gas Con., 5, 952–965, 2011.
Holloway, S. and Savage, D.: The potential for aquifer disposal of carbon dioxide in the UK, Energ. Convers. Manage., 34, 925–932, 1993.
Holz, M.: The Eo-Permian coal seams of the paraná basin in southernmost Brazil: an analysis of the depositonal conditions using sequence stratigraphy concepts, Coal Geology, 26, 141–163, 1998.
Holz, M.: Sequence stratigraphy of a lagoonal estuarine system – an example from the lower Permian Rio Bonito Formation, Paraná Basin, Brazil, Sediment. Geol., 162, 305–331, 2003.
Holz, M. and Carlucci, R.: Litoestratigrafia, estratigrafia de sequencias e evolução paleofisiográfica da zona de borda da Bacia do Paraná no Rio Grande do Sul durante o Eo-Permiano, in: Geologia do Rio Grande do Sul, edited by: Holz, M. and De Ros, L. F., CIGO UFRGS, 303–322, 2000.
Holz, M., Küchle, J., Philipp, R. P., Bischoff, A. P., and Arima, N.: Hierarchy of tectonic control on stratigraphic signatures: Base-level changes during the Early Permian in the Paraná Basin, southernmost Brazil, J. S. Am. Earth Sci., 22, 85–204, 2006.
IEA Greenhouse Gas R&D Programme (IEA GHG): CCS Site Characterisation Criteria, report number 2009/10, 2009.
Intergovernmental Panel on Climate Change (IPCC): Climate Change 2014: Synthesis Report. Contribution of Working Groups I, II and III to the Fifth Assessment Report of the Intergovernmental Panel on Climate Change, edited by: Core Writing Team, Pachauri, R. K., and Meyer, L. A., IPCC, Geneva, Switzerland, 151 pp., ISBN 978-92-9169-143-2, 2014.
Intergovernmental Panel on Climate Change (IPCC): Climate Change 2023: Synthesis Report. Contribution of Working Groups I, II and III to the Sixth Assessment Report of the Intergovernmental Panel on Climate Change, edited by: Core Writing Team, Lee, H., and Romero, J., IPCC, Geneva, Switzerland, 35–115, https://doi.org/10.59327/IPCC/AR6-9789291691647, 2023.
Jayasekara, D. W., Ranjith, P. G., Wanniarachchi, W. A. M., Rathnaweera, T. D., and Chaudhuri, A.: Effect of salinity on supercritical CO2 permeability of caprock in deep saline aquifers: an experimental study, Energy, 191, 116486, https://doi.org/10.1016/j.energy.2019.116486, 2020.
Karner, G. D. and Gamboa, L. A. P.: Timing and origin of the South Atlantic pre-salt sag basins and their capping evaporates, in: Evaporites Through Space and Time, edited by: Schreiber, B. C., Lugli, S., and Bąbel, M., Geological Society, London, Special Publications, 285, 15–35, 2007.
Karner, G. D., Driscoll, N. W., and Barker, D. H. N.: Synrift subsidence across the West African continental margin: The role of lower plate ductile extension, in: Petroleum Geology of Africa: New Themes and Developing Technologies, edited by: Arthur, T. J., Macgregor, D. S., and Cameron, N. R., Geological Society, London, Special Publications, 207, 105–125, 2003.
Ketzer, J. M., Holz, M., Morad, S., and Al-Aasm, I. S.: Sequence stratigraphic distribution of diagenetic alterations in coal-bearing, paralic sandstones: evidence from the Rio Bonito Formation (early Permian), southern Brazil, Sedimentology, 50, 855–877, 2003.
Ketzer, J. M., Iglesias, R., Einloft, S., Dullius, J., Ligabue, R., and de Lima, V.: Water-rock-CO2 interactions in saline aquifers aimed for carbon dioxide storage: experimental and numerical modeling studies of the Rio Bonito Formation (Permian), Southern Brazil, Appl. Geochem., 24, 760–767, 2009.
Kolenovic, I. and Saftic, B.: Geološko skladištenje ugljičnog dioksida, Rudarsko-geološko-naftni zbornik, 28, 9–22, 2014.
Kuchle, J. and Holz, M.: Aplicação da estratigrafia de sequencias para caracterização em multiescala de reservatórios no Grupo Guatá (Eopermiano da Bacia do Paraná) na região de São Gabriel – RS, Brasil, Pesquisas em Geociências, 28, 3–20, 2002.
Lavina, E. L. and Lopes, R. C.: A transgressao marinha do Permiano Inferior e a evoluçao paleogeografica do Super-Grupo Tubarao no Estado do Rio Grande do Sul, Paula Coutiana, 1, 51–103, 1987.
Lima, V. D., Einloft, S., Ketzer, J. M., Jullien, M., Bildstein, O., and Petronin, J. C.: CO2 Geological storage in saline aquifers: Paraná Basin caprock and reservoir chemical reactivity, Enrgy. Proced., 4, 5377–5384, 2011.
Maahs, R., Kuchle, J., Scherer, C. M. S., and Alvarenga, R. S.: Sequence stratigraphy of fluvial to shallow-marine deposits: the case of the early Permian Rio Bonito Formation, Paraná Basin, southernmost Brazil, Braz. J. Geol., 49, e20190059, https://doi.org/10.1590/2317-4889201920190059, 2019.
Maahs, R., Kuchle, J., Rodrigues, A. G., da Silva, T. F., González, M. B., and Erthal, F.: Paleoenvironmental reconstruction of the Permian coal beds in the Paraná Basin (Brazil): evidence from organic geochemical and sedimentological analyses, J. S. Am. Earth Sci., 128, 104484, https://doi.org/10.1016/j.jsames.2023.104484, 2023.
Maahs, R., Kuchle, J., Rodrigues, A. G., Trombetta, M. C., Alvarenga, R. S., Barili, R., and Freitas, W.: Three-dimensional geological modeling applied to multiscale heterogeneity of a reservoir analog: Paleocoastal deposits from the Rio Bonito Formation, Paraná Basin, Mar. Petrol. Geol., 167, 106930, https://doi.org/10.1016/j.marpetgeo.2024.106930, 2024.
Machado, C. X., Rockett, G. C., and Ketzer, J. M. M.: Brazilian Renewable Carbon Capture and Geological Storage Map: Possibilities for the Paraná Basin, Enrgy. Proced., 37, 6105–6111, 2013.
Machado, J. L. F. and Freitas, M. A. D.: Projeto mapa hidrogeológico do Estado do Rio Grande do Sul – Relatório final, 1. CPRM, 65 pp., 2005.
MapBiomas Brasil: Infrastructure Data, MapBiomas Brasil [data set], https://brasil.mapbiomas.org/en/dados-de-infraestrutura/, last access: 20 March 2024.
Maskell, A., Scoth, P. M., Buimaman, I., and Bickle, M.: A siltstone reaction related to CO2 and sulfur-bearing fluids: integrating quantitative elemental mapping with reactive transport modelling, Am. Mineral., 103, 314–323, 2018.
Milani, E. J.: Evolução tectono-estratigráfica da Bacia do Paraná e seu relacionamento com a geodinâmica fanerozóica do Gondwana sul-ocidental. 2 v. Tese (Doutorado) – Universidade Federal do Rio Grande do Sul, Porto Alegre, 1997.
Milani, E. J. and Zalán, P. V.: The Geology of Paleozoic Cratonic Basins and Mesozoic Interior Rifts of Brazil, AAPG Int. Conf. & Exhibition, 8–11 November 1998, Rio de Janeiro, Brazil, Search and Discovery Article no. 90933, 1998.
Milani, E. J., Faccini, U. F., Scherer, C. M., Araújo, L. M., and Cupertino, J. A.: Sequences and stratigraphic hierarchy of the Paraná Basin (Ordovician to Cretaceous), Southern Brazil, Boletim Instituto de Geociências Universidade de São Paulo, Série Científica, 29, 125–173, 1998.
Milani, E. J., Melo J. H. G., Souza, P. A., Fernandes, L. A., and França, A. B.: Bacia do Paraná, Bol. Geociencias Petrobras, 15, 265–287, 2007.
Mohriak, W. U.: Bacias Sedimentares Da Margem Continental Brasileira, in: Geologia, Tectônica e Recursos Minerais do Brasil, edited by: Bizzi, L. A., Schobbenhaus, C., Vidotti, R. M. & Gonçalves, J. H., Companhia de Pesquisas de Recursos Minerais, Brasília, 87–165, ISBN 85-230-0790-3, 2003.
Moreira, J. R., Romeiro, V., Fuss, S., Kraxner, F., and Pacca, S. A.: BECCS potential in Brazil: Achieving negative emissions in ethanol and electricity production based on sugar cane bagasse and other residues, Appl. Energ., 179, 55–63, 2016.
Neves, I. d. A., Lupinacci, W. M., and Silva, C. G.: A new geological model based on a seismic-stratigraphic analysis of the eastern Inhambú Oil Field, onshore Espírito Santo Basin, Brazil, Braz. J. Geophys., 36, 1–16, 2018.
Oelkers, E. H., Gíslason, S. R., and Matter, J.: Mineral Carbonation of CO2, Elements, 4, 333–337, 2008.
Oliveira, S. B., Weber, N., Yeates, C., and Tassinari, C. C. G.: Geological screening for onshore CO2 storage in the Rio Bonito formation, Paraná Basin, Brazil, J. Maps, 19, 2171817, https://doi.org/10.1080/17445647.2023.2171817, 2023.
Orita, G. K. L. and Da Cruz, V. G. P.: Captura e armazenamento de CO2: Uma revisão das tecnologias existentes, carbonatação in situ de basaltos e avaliação do potencial da Formação Serra Geral como reservatório de CO2, Geociências, 41, 779–795, https://doi.org/10.5016/geociencias.v41i03.16760, 2022.
Pantopoulos, G., Orita, G. K. L., Armelenti, G., Althaus, C. E., Kuchle, J., Scherer, C. M. S., Rodrigues, A. G., and De Ros, L. F.: Depositional conditions at the Aptian pre-salt margins: evidence from quantitative petrography and textural analysis of the Mucuri Member, Espírito Santo Basin, Brazil, Petrol. Geosci., 27, petgeo2020-112, https://doi.org/10.1144/petgeo2020-112, 2021.
Paulipetro: Geologia da Bacia do Paraná – Reavaliação da potencialidade e prospectividade em Hidrocarbonetos, Consórcio CESP/IPT, São Paulo, Brasil, 198 pp., OCLC 14944178, 1982.
Prevedel, B., Wohlgemuth, L., Henninges, J., Krüger, K., Norden, B., Förster, A., and the CO2SINK Drilling Group: The CO2SINK Boreholes for Geological Storage Testing, Sci. Dril., 6, 32–37, https://doi.org/10.2204/iodp.sd.6.04.2008, 2008.
Rackley, S. A.: Carbon Capture and Storage; 2nd edn., Butterworth-Heinemann, Cambridge, MA, USA, ISBN 978-0-12-812041-5, 2017.
Raza, A., Gholami, R., Rezaee, R., Bing, C. H., Nagarajan, R., and Hamid, M. A.: CO2 storage in depleted gas reservoirs: A study on the effect of residual gas saturation, Petroleum, 4, 95–107, 2018.
Rockett, G. C., Machado, C. X., Ketzer, J. M. M., and Centeno, C. I.: The CARBMAP project: Matching CO2 sources and geological sinks in Brazil using geographic information system, Enrgy. Proced., 4, 2764–2771, 2011.
Scherer, C. M. S., Reis, A. D., Horn, B. L. D., Bertolini, G., Lavina, E. L. C., Kifumbi, C., and Goso Aguilar, C.: The stratigraphic puzzle of the permo-mesozoic southwestern Gondwana: The Paraná Basin record in geotectonic and palaeoclimatic context, Earth-Sci. Rev., 240, 104397, https://doi.org/10.1016/j.earscirev.2023.104397, 2023.
Sistema de Estimativas de Emissões e Remoções de Gases de Efeito Estufa (SEEG): Map of CO2 emissions by Brazilian state, https://seeg.eco.br/en/home/#emissoes, last access: 5 November 2024.
Szatmari, P.: Habitat of petroleum along the South Atlantic margins, in: Petroleum Systems of South Atlantic Margins, Tulsa, edited by: Mello, M. R. and Katz, B. J., AAPG Memoir, 73, 69–75, 2000.
van der Meer, L. G. H. and Yavuz, F.: CO2 storage capacity calculations for the Dutch subsurface, Enrgy. Proced., 1, 2615–2622, 2009.
Vieira, R. A. B.: Análise Estratigráfica e Evolução Paleogeográfica da Seção Neoaptiana na Porção Sul da Plataforma de São Mateus, Bacia do Espírito Santo, Brasil, Dissertation (Master's), Universidade Federal do Rio Grande do Sul, Porto Alegre, 1998.
Vieira, R. A. B., Mendes, M. P., Vieira, P. E., Costa, L. A. R., Tagliari, C. V., Bacelar, L. A. P., and Feijó, F. J.: Bacias do Espírito Santo e Mucuri, Boletim de Geociências da Petrobrás, 8, 191–202, 1994.
Weber, N., de Oliveira, S. B., Cavallari, A., Morbach, I., Tassinari, C. C. G., and Meneghini, J.: Assessing the potential for CO2 storage in saline aquifers in Brazil: Challenges and Opportunities, Greenh. Gases, 14, 319–329, 2024.
White, I. C.: Relatório sobre as Coal Measures e rochas associadas ao sul do Brasil: Relatório final da Comissão dos Estudos das Minas de Carvão de Pedra do Brasil, Rio de Janeiro, Brasil, 1908.
Wildenborg, T., Chadwick, A., Deflandre, J., Eiken, O., Mathieson, A., Metcalfe, R., Schmidt Hattenberger, C., and Wollenweber, J.: Key messages from active CO2 storage sites, Enrg. Proced., 37, 6317–6325, 2013.
Zalan, P. V., Wolff, S., Conceição, J. C. J., Marques, A., Astolfi, M. A. M., Vieira, I. S., Appi, V. T., and Zanotto, O. A.: Bacia do Paraná, in: Origem e Evolução de Bacias Sedimentares, Petrobras, Rio de Janeiro, 135–169, CDD 551.0981.551.8, 1990.