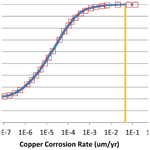
Assessment of canister degradation for the encapsulation of spent nuclear fuel: Key research issues encountered in recent regulatory reviews and government decision making in Sweden
Bo Strömberg
Elena Calota
Jinsong Liu
Michael Egan
Regulatory review of a licence application for construction and operation of a geological repository for radioactive waste should not only be based on information provided by the applicant but also on viewpoints brought up by stakeholders and decision makers. In the Swedish Radiation Safety Authority's (SSM) licensing review of a spent fuel repository at the Forsmark site, the main concerns brought up in court hearings, from national consultations and by the Swedish government were focused around the long-term protective capacity of the copper canisters with cast iron inserts. The most debated canister degradation mechanisms were anoxic corrosion of copper (in oxygen gas free water), localised sulphide corrosion (pitting corrosion and stress corrosion cracking), the influence of gamma radiation, and strain hardening of the canister cast iron insert. The main question addressed by SSM is not whether such effects can be ruled out altogether, but rather their potential extent and influence on canister integrity during a sufficiently long period in the repository environment. Constraints are mainly based on rock properties and associated hydrological and geochemical factors known from site investigation and knowledge about the protective capability of the buffer barrier. The key factors are in general well characterised and cover for instance sulphide availability, groundwater flow, mass transfer rates and expected mechanical loading on canisters in the repository environment. The canister corrosion/degradation mechanisms reviewed in this paper are judged by SSM to have a small or limited importance for demonstration of regulatory compliance, taking into account the characteristics of the site, the function of the buffer, and the margins provided by the significant thickness and mechanical strength of the proposed canister.
- Article
(1138 KB) - Full-text XML
- BibTeX
- EndNote
Geological disposal of spent nuclear fuel has the ultimate purpose of ensuring safety for future generations and that exposure to radiation-related risks for those living in close proximity to a sealed disposal facility is below regulatory targets and minimized as far as reasonably practicable. An implementer/repository developer/applicant has the responsibility to design, construct and operate the facility in such a way that these objectives can be met. The different steps consist of developing a repository concept including the engineered barriers, selecting and investigating a suitable candidate site, and showing with practical demonstration that the repository and its components can be constructed as intended. The steps also include demonstrating safety, which is especially important for building necessary confidence for designers, decision makers and stakeholders. The role of a national regulatory body, such as the Swedish Radiation Safety Authority (SSM) in Sweden, is to define regulatory requirements, and to verify through thorough regulatory review and analysis that such requirements will be met by the implementer's proposals.
The plans for a deep geological repository for spent nuclear fuel in the Swedish crystalline bedrock started more than 40 years ago. Long-lived containment in copper canisters was suggested at an early stage as a key safety function. Apart from the canister, a surrounding bentonite clay barrier and the bedrock comprise the other two main barriers of the KBS-3 repository concept used in the Swedish license application. The Forsmark and Laxemar sites in Sweden were thoroughly evaluated as candidate sites in between 2002–2008. In 2011, the Swedish Nuclear Fuel and Waste Management Company (SKB) submitted a licence application for construction and operation of a repository based on the KBS-3 concept at the Forsmark site. Long-term safety was addressed in the SR-Site report as one of the supporting documents to the application (SKB, 2011).
In January 2022, the Swedish government approved the application for construction and operation of a repository for spent nuclear fuel at the Forsmark site. Leading up to this decision, and after completion of the main regulatory review phase in 2018, SSM reported to the government on several occasions on various degradation mechanisms for the canister's copper shell and cast iron insert, both of which had recently been debated (SSM, 2019, 2021a, b). In particular, decision makers were concerned that any new information would raise new questions regarding the suitability of the canister design. The concerns were largely raised by independent researchers from Sweden and Finland (e.g. Szakalos and Leygraf, 2019), and a number of environmental organisations. Those mechanisms were in particular:
-
Anoxic corrosion of copper
-
Localised sulphide corrosion of copper (pitting corrosion, stress corrosion cracking)
-
Radiation induced effects
-
Strain hardening of cast iron
In this paper, we summarize the scientific basis of the degradation mechanisms, and provide a discussion of their overall importance in the assessment of canister failure and consequently long-term radionuclide releases from the repository, which SSM presented to the Swedish government in late 2021.
The method used here for the overall assessment of canister issues in the late stages of the licensing process is based on three steps: (1) an overview of mechanistic process understanding based on experiments as well as the theoretical basis; (2) the identification of factors in the repository environment and the multi-barrier system that have or may have important safety implications; and (3) a summary of calculations or supporting arguments to evaluate process-significance for repository long-term performance. The main role of SSM is to judge compliance based on material provided by the applicant. Inputs from national consultation and independent calculations conducted on behalf of SSM nonetheless represent additional information of relevance for judging critical compliance issues. The main regulatory requirement of relevance in the context of copper canister lifetime is that of limiting radionuclide releases from the sealed repository such that a risk of 10−6 per year for harmful effects due to radiation dose for a representative person in the most exposed group is not exceeded. The first 100 000 years constitute the main compliance period during which this quantitative criterion is applied (SSM, 2008).
The anoxic copper corrosion process in oxygen gas free water has been proposed by the engaged stakeholders to be a key objection to the choice of copper as a corrosion barrier (e.g. Szakalos and Leygraf, 2019). Two variants of this reaction are:
The process in its first variant (Eq. 1) involves formation of cuprite as the final stable corrosion product. This reaction is not excluded from occurring; however the hydrogen partial pressure at chemical equilibrium can be readily determined, based on properties of known phases, to be vanishingly small at 10−9 bar. The second variant (Eq. 2), involving the formation of CuOH or a similar phase, has however been much debated based on the results of experiments with hydrogen evolution measurements. A corresponding hydrogen partial pressure of 10−3 bar was suggested (Szakalos et al., 2007). This corrosion product phase was associated with a presumed decreased stability range of copper, but in spite of several attempts by others, it has so far has never been isolated and positively identified (e.g. Ottosson et al., 2017). Even if it were to be formed, however, the postulated influence on corrosion properties seems unlikely when compared with the pure monovalent oxide phase. SSM thus concluded that the case with the second variant is highly unlikely to be significant for the question of canister corrosion (SSM, 2019).
Against the background of the applicant's safety assessment placing greatest emphasis on the consequences of general sulphide corrosion (e.g. Eq. 3), challenges raised during the consultation were concerned with associated forms of localised corrosion of copper such as pitting corrosion or stress corrosion cracking (SCC). In all these cases, the degradation processes are related to the long-term availability of sulphide ions in the repository environment. The most important chemical reaction can conservatively be represented as:
Traditionally, different forms of localised corrosion have been associated with the formation of a passivating corrosion product layer (Frankel, 1998), in this case copper sulphides, on the metal surface. The layer may then be disturbed locally by mechanical forces (SCC) or by chemical factors (pitting) to initiate a more severe form of corrosion. In the absence of a passivating sulphide layer, sulphide ions reaching the canister would be consumed by an essentially evenly distributed general corrosion and could thus not contribute to localised corrosion. In the proponent's original application, it was asserted that only porous and non-protective films would form (SKB, 2011). Later complementary work however suggests that for high sulphide loadings a more dense and protective film may form under certain condition, which could generate a form of pitting termed microgalvanic corrosion (SKB, 2019). For lower loading however, the film was still considered too porous to be protective. In an independent experimental study initiated by SSM, it was confirmed that a passivating film could form on pure copper even at lower sulphide loadings, but for some presently unknown reason the tendency of pitting was observed only on pure copper but not on the OFP-copper (containing 50 ppm phosphorus) intended to be used for the canister (Macdonald et al., 2016).
A potential stress corrosion cracking (SCC) of copper is by definition a more complex form of corrosion due to the dependency on not only mass transfer and chemical conditions but also the associated mechanical loading conditions. An SCC mechanism linked to sulphide would be dependent on simultaneous occurrence of tensile stresses on small sections of the canister and a persistent supply of sulphide ions to the same canister region. Various studies of SCC based on copper and sulphide have been conducted. Anything resembling fracture formation in SCC experiments, either in studies conducted by SKB, SSM or others, have however so far not been detected for sulphide concentrations lower than 1 mM (Becker et al., 2020).
A third theme which has been debated is the effect of the early gamma radiation field outside the canister. As shown in various experiments, radiolytic oxidants will contribute to corrosion of copper during the early stage of repository evolution (Björkbacka et al., 2017). The direct accumulated corrosion effects can only be minor based on the shielding provided by the canister thickness and the half-lives of the radionuclides that contribute to dose, but it has been proposed that the copper bulk material properties could be affected (Szakalos and Leygraf, 2019). If the material becomes more brittle it would be more susceptible to mechanically-induced failures such as SCC. Similar arguments have also been raised in relation to possible hydrogen uptake, due to either radiation effects or corrosion, and consequent potential hydrogen embrittlement of copper.
The fourth theme is completely unrelated to copper and concerns only the cast iron insert of the disposal canister. The basis for this topic is an experimental study of strain hardening, which could reduce the load bearing capacity of the insert. A number of experiments were conducted at different temperatures (room temperature, 100, 200, 300, 400 ∘C), which showed a significantly increased yield strength for “aged” test rods that had previously been deformed (1 %, 2 % and 3 % strain), in comparison with reference rods (Björklund, 2021). It was suggested that this potentially deleterious effect had not previously been sufficiently accounted for in the applicants' design and safety analysis.
The main environment conditions controlling copper corrosion are expected to be groundwater chemistry, availability of water conducting features and groundwater flow, as well as the mass transfer properties of the bentonite buffer. The overall groundwater chemical conditions also provide a basis for assessment of alteration and erosion of the buffer. The key parameters for corrosion of copper and erosion of bentonite, respectively, are redox potential after depletion of initial oxygen, and salinity determined mainly by groundwater chloride and base cation content.
The in situ rate of the anoxic corrosion discussed in the section above can be estimated based on the product of the thermodynamic driving forces and the repository mass transfer rates through the buffer and near-field crystalline rock. Negligible rates are justified because both these factors are independently very small. Reducing groundwater is expected to contain small quantities of hydrogen, which would further stifle or inhibit the reaction, but site data on current hydrogen content from site investigations is sparse. It should also be pointed out that the amount of residual oxygen from the repository construction phase is much too limited to have a significant corrosion effect. Moreover, the potential for future inflow of atmospheric oxygen to a sealed repository, although not fully ruled out, is an unlikely risk factor due to the limited rates of mass transfer and to the vast reservoir of mineral reductants such as biotite and pyrite in the bedrock (SSM, 2018).
The above-mentioned anoxic corrosion process has also been debated in the context of the LOT long-term field experiments in the Äspö hard rock laboratory (SKB, 2020). It has been suggested that detected corrosion during these experiments should be attributed to the anoxic reaction, and thus provides evidence of its significance. However, SSM concluded in its review that the observed extent of corrosion after 20 years' exposure is similar to that found in LOT experiments curtailed after a duration of only a few years, and that the identified corrosion products are consistent with corrosion occurring under oxidising conditions (Eq. 4) at the start of the experiment (SSM, 2021).
A new result from the 20 year LOT experiment, by contrast with those of shorter duration, is nevertheless that reasonably convincing evidence for the onset of sulphide corrosion could be found in part of the experiment (SKB, 2020), while other parts of the experiment may still be relatively oxidising due to remaining Cu(II) even long after the depletion of initial oxygen.
Regarding the extent of sulphide corrosion, regardless of whether this was in the form of general or localised corrosion, it is considered that any remaining or generated sulphide in the void space of the repository would be quickly depleted, and that continued corrosion of the canister by sulphide would require transport routes to the specific deposition hole to be established. Three main routes can be considered:
-
Diffusion of gaseous sulphide through the buffer from the backfilled tunnel above the deposition hole
-
Diffusion of aqueous sulphide through the buffer from the backfilled tunnel above the deposition hole
-
Sulphide in groundwater from hydraulically conductive fractures intersecting a deposition hole
Scooping calculations suggest that the only realistic potential route for a rapid supply of sulphide to a deposition hole during the early stages of repository evolution, when the buffer is unsaturated, is the gaseous transport route (Lilja et al., 2013), in particular because a significant fraction of deposition holes at the Forsmark site are expected to have long resaturation times. The corresponding aqueous route can be shown to be insignificant since aqueous diffusion is about five orders of magnitude slower than the corresponding diffusion in the gaseous phase. Microbial sulphate reduction (MSR) is most likely the dominant source for any transfer of sulphide from the backfill tunnel (represented below by the consumption of dissolved methane gas):
After resaturation of the buffer is completed, slow diffusion in combination with the significant thickness of the buffer section above the canister ensures that the only conceivable route for further sulphide transport and corrosion is through groundwater flow in fractures intersecting a deposition hole. The mass flux of sulphide towards a canister surface in this case will vary considerably depending on the specific properties of a deposition hole, with the highest potential corrosion rates if an unusually high content of groundwater sulphide were to coincide with a deposition hole being intersected by a highly water-conducting fracture. Even so, an intact buffer would prevent any advective transport in the deposition hole thus severely restrict sulphide mass-transfer. With transport of sulphide towards a canister being limited to very slow rates under such conditions, canister corrosion will be slow even considering unfavourable combinations in the bedrock.
Assessment of any failure mode related to mechanical properties of either copper or cast iron is based on the two possible canister loading conditions in the repository environment, namely isostatic loading associated with buffer swelling pressure and groundwater pressure, and shear loading from rapid rock movements associated with large earthquakes (SKB, 2011). Although both these cases are accounted for in the canister design basis, the associated significant differences in potential risk relevance must be considered. The former type of loading conditions in principle concerns all canisters and relatively early time periods in the post-closure evolution of the repository, while the latter period concerns a much smaller number of canisters and the low probability event of a large earthquake in close proximity to the repository, mostly associated with future glaciation events and often referred to as post-glacial faulting. The isostatic loading based on combined buffer swelling pressure and groundwater pressure at repository depth is generally constrained to about 14 MPa, while the design of the insert must account for much larger loads around 50 MPa for a time in the future when the repository site could potentially be covered by a thick ice sheet. The relevant shear loading conditions are based on rock movement propagated from a primary fault through the fracture network, with shear failure of a canister being associated with seismic events ≥ M 5.
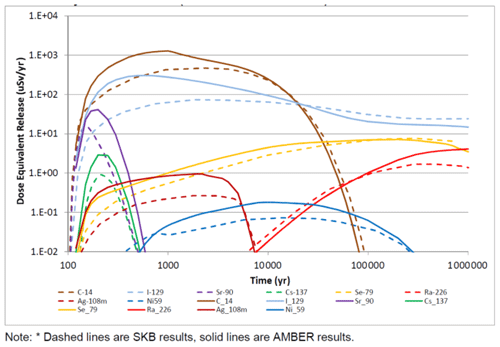
Figure 1Predicted future radiation dose for a representative individual in the most exposed group for the first 1 million years based on the hypothetical assumption that all 6000 disposed canister are failed already at the time of repository closure. The solid lines are calculations conducted on behalf of SSM (Penfold, 2014) and the dotted lines are from the applicant's safety assessment (SKB, 2011).
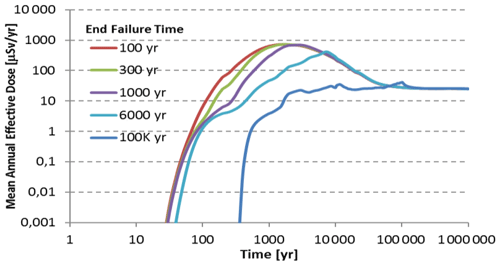
Figure 2Predicted future radiation dose for a representative individual in the most exposed group for the first 1 million years calculated in order to illustrate the significance of an early hypothetical failure mechanism. The failures are assumed to be evenly distributed during five different time periods of different duration (Pensado, 2017).
The significance of any remaining uncertainty regarding canister degradation can ultimately be addressed in the context of the systems perspective and repository licensing by considering constraints on radionuclide transport provided by the other two barriers, namely the bentonite buffer and the host rock. Figure 1 shows a dose calculation based on the limiting and most pessimistic assumption that all canisters are failed at the outset and that long-term safety therefore relies solely on the other two barriers. SSM's calculation and the corresponding one by the applicant show close correspondence with peak annual doses close to those associated with the background radiation (peak annual doses around 1 mSv). Figure 2 shows an alternative representation of a hypothetical early failure mechanism, in which canister failures are assumed to be evenly distributed over time intervals of different duration. As expected, the calculated peak annual dose decreases considerably with increasing length of the failure time interval. These hypothetical cases are illustrative only, and should not be taken to suggest that canisters can be expected to fail early or that the canister performance is considered to be of minor significance.
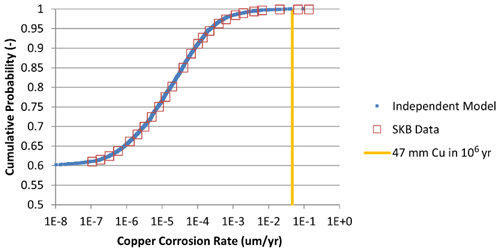
Figure 3Cumulative distribution for predicted copper corrosion rates in the repository environment for a fixed relatively high sulphide concentration (10−5 M) and based on the assumption that the buffers in all deposition holes are substantially failed, thereby facilitating advective conditions in the deposition holes and hence more rapid corrosion. The distribution is based on the hydraulic characteristics of the full ensemble of deposition holes of which 60 % are expected to have no fracture interconnections. The red squares represent the calculations conducted by the applicant (SKB, 2011) and the blue dots calculations conducted on behalf of SSM (Benbow et al., 2015). The yellow line represent the rate associated with failures in 1 million years. This means that it is only the two data points to the right of this yellow line that represent repository conditions facilitating canister corrosion failure.
No canister failures are expected on time frames relevant to assessing quantitative compliance with the regulatory risk criterion so long as: (i) only general corrosion of the copper canisters takes place, (ii) the bentonite buffers remain intact and are not significantly affected by erosion, (iii) the measured distribution of sulphide levels in groundwater (approx. 10−7 to 10−5 M) apply indefinitely and (iv) the assigned groundwater flow rates are representative even for the most exposed deposition holes. Figure 3 shows an alternative hypothetical case addressed by SSM, based on the assumption of a fixed high sulphide concentration (10−5 M) and buffers that are substantially eroded in all deposition holes. In this case, only a handful of the 6000 canisters are projected to fail after 1 million years. The reduction in canister lifetimes for this hypothetical case is based on the prior assumption that the buffer has been substantially eroded to allow advection transport of sulphide in groundwater near canister surfaces. In practice, the predicted time until advective conditions are established in any deposition hole, thereby enabling more rapid corrosion, is itself a large fraction of the compliance period. The potential for reduction of the nominal 50 mm thickness of the canister's copper shell due to manufacturing imperfections giving rise to surface defects, and the millimetre-scale corrosion by initial oxygen as well as supply of gaseous sulphide, are relatively minor in the long-term context but must also be taken into account.
The mass balance/transfer considerations briefly discussed above also provide a reasonable basis to estimate pitting corrosion according to the ratios of reasonable pit propagation in relation to the extent of general corrosion. Empirical knowledge suggests pitting ratios of about 4–25 (King et al., 2010). Even the upper value of this range would not significantly influence the failure of canisters in deposition holes with an intact buffer, while the projected timing and extent of eroded buffer conditions mean that the implications of pitting corrosion for compliance with safety requirements are small. If one also considers the probable circumstance that only those deposition holes that are associated with relatively high sulphide loading are likely to be relevant for the formation of passivating copper sulphide film, the effect is even less pronounced (Pensado and Stothoff, 2019). In order for reproductions of such failure rate calculation cases by the regulator to have significant review value and be a useful basis to assess canister failure rates, they need to be linked to reviews of utilised data and assumptions in the modelling, for instance regarding groundwater sulphide levels (Bath, 2014), mass transfer effects in and near deposition holes (Stothoff and Manepally, 2013) and groundwater flow rates (Geier, 2014), as well as exploring the significance of alternative model assumptions (Benbow et al., 2015; Pensado and Stothoff, 2019). The associated step for the dose calculations in Figs. 1 and 2 is considered outside the scope of this paper.
The stress corrosion cracking topic is not easily addressed by such a mass balance approach since the sulphide flux level that could potentially cause a significant canister damage is difficult to ascertain. Even if the existence of an SCC mechanism linked to sulphide has been questioned, if it were to exist it would be dependent on simultaneous occurrence of tensile stresses on small sections of the canister and a persistent supply of sulphide ions to the same canister region. The most relevant time scale and location for such conditions would be the early period of buffer swelling and associated loading of the canister, as well as sulphide supply to the canister upper lid surface area due to gaseous diffusion from the backfill. The time window for such an effect is, however, relatively small since build-up of swelling pressure within the buffer is also associated with the closing of the gaseous path. The most appropriate exclusion strategy with the current level of knowledge is probably to avoid any buffer and backfill material that contains significant reactive organic matter, potentially contributing to MSR.
The radiation induced effects on the material properties of copper as well as cast iron different from the factors considered above in the sense that their implications are hardly dependent on the rock conditions and repository environment, and can thus only be appropriately addressed in terms of canister design specifications. The additional tests that were conducted to specifically address this concern for copper were based on pure metal samples and not on OFP copper intended to be used for the copper canister. Even if radiation induced effects are expected to be minor and not significantly affected by copper quality, SSM recommended additional tests to be conducted in the future based on representative canister materials.
The concept and implications of strain hardening concern the cast iron insert and are assessed to be exclusively linked to effects associated with shear movement in the near-field rock and large earthquakes, most likely having the potential to affect only a small number of canisters far into the future. The reason for this is that the insert has been designed based on the condition that the normal and expected isostatic loading on all canisters in the repository will not be a cause for a permanent deformation. Furthermore, potential concerns linked to strain hardening are relevant only for the case where an insert that has previously been permanently deformed is subsequently deformed again, i.e. if a second large earthquake affects the same canister position. Since a large earthquake in close vicinity to the repository site is by itself an improbable event with a return period that is of the same approximate order of magnitude as the regulatory compliance period, strain hardening would be an unlikely candidate for significant influence in the risk analysis. Nevertheless, further exploration of this issue is considered appropriate to ensure the completeness of the assessment.
External viewpoints provided during the Swedish spent nuclear fuel repository licensing process have to a large extent focused on the supposition that the protective capacity of the copper canister will be much poorer than is assumed in the applicant's safety analysis. Although the specific reasons for early canister failures postulated by independent researchers have varied somewhat over the years, the proposed end result has been canister life time distributions that are several orders of magnitude shorter (Szakalos and Leygraf, 2019) than those presented in the proponent's safety case (SKB, 2011). In this paper, we have considered a range of issues related to this topic for which the Swedish government requested additional assessment from SSM prior to their licensing decision.
The regulatory review for addressing these concerns has been based on two different approaches: (i) accepting the premise of an assumed poor canister performance and exploring its implications for radiological safety; and (ii) addressing the scientific basis for postulated accelerated canister degradation mechanisms, together with the repository environmental conditions that influence their extent (typically determined by the rock and buffer barriers). The former approach includes an extensive review of radionuclide transport retardation as a repository safety function, which suggests post closure dose levels – even when all canisters are assumed to be failed relatively early or even at the outset – below or around those from background radiation for the most exposed group living in close proximity to the repository foot print (see Figs. 1 and 2).
The second approach starts with an evaluation of all experimental work conducted both by the proponent (King et al., 2010), and the critics (e.g. Szakalos et al., 2007) as well as SSM's own external experts (e.g. Macdonald et al., 2016). The findings from these studies have then been coupled with a review of available knowledge regarding the repository environment and its long-term evolution related, taking into consideration factors such as groundwater flow, groundwater chemistry and loading conditions on the canister (SKB, 2011). The implications of specific mechanisms and processes related solely to corrosion (anoxic corrosion in oxygen gas free water, pitting corrosion due to groundwater sulphide) can be assessed based on arguments and evidence related to thermodynamics, mass transfer rates as well as mass balance. However, processes involving mechanical aspects of canister performance (stress corrosion cracking, radiation induced effects, strain hardening of cast iron) also have to be analysed taking into account more stringent requirements on barrier manufacturing process, results from materials testing, and/or application of safety margins to account for any remaining uncertainties. Hypothetical limiting and unrealistic calculation cases have also been used to explore the sensitivity of model assumptions to the probability and extent of canister failures (see e.g. Fig. 3).
There is no doubt that the scientific debate on canister degradation processes has constructively contributed to various insights related to long-term canister and repository performance. Nevertheless, the authors of this paper have not been able to find any compelling arguments and analyses that support that the assertion that canister life times will be far shorter than those presented in the proponent's safety case. New information related to the above-mentioned processes may still emerge in the future and it cannot be entirely ruled out that new issues will emerge that could have an impact on the assessed performance of engineered barriers, the surrounding bedrock and the future biosphere conditions. It is thus necessary to continue with a long-term research program and to update the safety assessment on a regular basis.
A number of proposed canister degradation mechanisms have been the topic of a debate on the suitability of the KBS-3 copper canister for disposal of spent nuclear fuel. SSM has addressed the scientific basis and potential importance of these mechanisms in the repository environment during the licensing of a final repository for spent nuclear fuel in Sweden. They include anoxic corrosion (in oxygen gas free water) and localised sulphide corrosion of copper (pitting corrosion and stress corrosion cracking), as well as potential long-term degradation of material properties due to radiation-induced effects and strain hardening. A range of laboratory and field investigations conducted during several decades indicates that such mechanisms may exist under certain conditions and must therefore be accounted for. However, combining this knowledge with review of available information about the repository environment and its evolution, as well as the function of other components of the disposal system, supports the licence applicant's contention that they are not impediments in the context of achieving long-term safety objectives. Some degree of uncertainty related to evolution of repository conditions is unavoidable and degradation mechanisms are difficult to study under fully representative conditions due to the long time scales. Nevertheless, the significant margins provided by the thickness of the copper corrosion barrier as well as the mechanical strength of the integrated canister contribute to providing the necessary conditions for the long-term safety demonstration.
Calculations presented in Figs. 1, 2 and 3 have been conducted using AMBER, GoldSim and Excel, respectively. AMBER is a commercially available compartment modelling software marketed by the Quintessa company (https://www.quintessa.org/software/AMBER, Quintessa, 2014). GoldSim is a commercially available simulation platform marketed by the GoldSim technology group (https://www.goldsim.com/Web/Products/GoldSim/Overview/, GoldSim Technology Group, 2016).
Description of how calculations were conducted including data usage is described in the following technical notes and partially also in associated references: Fig. 1: https://www.stralsakerhetsmyndigheten.se/publikationer/rapporter/avfall--transport--fysiskt-skydd/2014/201455/ (Penfold, 2014); Fig. 2: https://www.stralsakerhetsmyndigheten.se/publikationer/rapporter/avfall--transport--fysiskt-skydd/2017/201715/ (Pensado, 2017); Fig. 3: https://www.stralsakerhetsmyndigheten.se/publikationer/rapporter/avfall--transport--fysiskt-skydd/2015/201549/ (Benbow et al., 2015).
BS is the main author and has addressed overall safety assessment context as well as corrosion modelling in particular based on groundwater chemistry and other site information. EC has addressed review issues related to canister materials properties. JL has addressed canister performance issues based on buffer properties and their long-term evolution. ME has been project leader for the review assignment and has addressed the overall strategy for regulatory compliance assessment.
The contact author has declared that none of the authors has any competing interests.
Publisher's note: Copernicus Publications remains neutral with regard to jurisdictional claims made in the text, published maps, institutional affiliations, or any other geographical representation in this paper. While Copernicus Publications makes every effort to include appropriate place names, the final responsibility lies with the authors.
This article is part of the special issue “European Geosciences Union General Assembly 2023, EGU Division Energy, Resources & Environment (ERE)”. It is a result of the EGU General Assembly 2023, Vienna, Austria, 23–28 April 2023.
The authors wish to thank the SSM external experts who contributed to the regulatory review of the issues addressed in this paper, as well as many other issues. Steven Benbow, Osvaldo Pensado and James Penfold are in particular acknowledged for efforts involving engineered barrier integrity modelling work.
This paper was edited by Michael Kühn and reviewed by Axel Liebscher and one anonymous referee.
Bath, A.: Independent assessment of groundwater sulphide content in the long-term, Swedish Radiation Safety Authority, SSM Technical Note, 2014, 48, 2014.
Becker, R., Forsström, A., Yagodzinskyy, Y., Hänninen, H., and Heikkilä, M.: Sulphide-induced stress corrosion cracking and hydrogen absorption in copper exposed to sulphide and chloride containing deoxygenated water at 90 ∘C, SSM Research, 2020, 01, Strålsäkerhetsmyndigheten, 2020.
Benbow, S. J., Metcalfe, R., and Burrow, J.: Independent Modelling of Engineered Barrier Evolution and Coupled THMC: Canister Corrosion Calculations in SR-Site, Swedish Radiation Safety Authority, SSM Technical Note, 2015, 49, https://www.stralsakerhetsmyndigheten.se/publikationer/rapporter/avfall--transport--fysiskt-skydd/2015/201549/ (last access: 24 Octobe 2023), 2015.
Björkbacka, Å., Johnson, C. M., Leygraf, C., and Jonsson, M.: Radiation induced corrosion of copper in humid air and argon atmospheres, J. Electrochem. Soc., 164, C201–C206, 2017.
Björklund, V.: The effects of static strain aging on the mechanical performance of nodular cast iron, Master's Thesis, Aalto University, 2021.
Frankel, G. S.: Pitting Corrosion of Metals: A Review of the Critical Factors, J. Electrochem. Soc., 145, 2186–2198, 1998.
Geier, J.: Assessment of flows to deposition holes – main review phase, Swedish Radiation Safety Authority, SSM Technical Note, 2014, 05, 2014.
GoldSim Technology Group: GoldSim Version 11, Seattle, Washington, USA, https://www.goldsim.com/Web/Products/GoldSim/Overview/ (last access: 26 October 2023), 2016.
King, F., Lilja, C., Pedersen, K., Pitkänen, P., and Vähänen, M.: An update of the state-of-the-art report on the corrosion of copper under expected conditions in a deep geologic repository, Updated 2011-10, SKB TR-10-67, Swedish Nuclear Fuel and Waste Management Co, 2010.
Lilja, C., Sellin, P., and Hedin, A.: Svar till SSM på begäran om komplettering rörande grundvattenkemi på kort och medellång sikt, 2014-07-03, SKBdoc 1437441, Svensk Kärnbränslehantering AB, 2013 (in Swedish).
Macdonald, D. D., Mao, F., Dong, C., and Sharifi-Asl, S.: Measurements of Parameter Values for Predicting Corrosion Phenomena on Copper in Swedish HLNW Repositories, SSM Report 2016:30, Swedish Radiation Safety Authority, 2016.
Ottosson, M., Boman, M., Berastegui, P., Andersson, Y., Hahlin, M., Korvela, M., and Berger, R.: Copper in ultrapure water, a scientific issue under debate, Corrosion Science, 122, 53–60, 2017.
Penfold, J.: Further Reproduction of SKB's Calculation Cases and Independent Calculations of Additional “What If?” Cases, Swedish Radiation Safety Authority, SSM Technical Note, 2014:55, https://www.stralsakerhetsmyndigheten.se/publikationer/rapporter/avfall--transport--fysiskt-skydd/2014/201455/ (last access: 24 October 2023), 2014.
Pensado, O.: Radionuclide release rates associated with bounding cases featuring relatively early canister failures in a spent fuel repository, Swedish Radiation Safety Authority, SSM Technical Note 2017:15, https://www.stralsakerhetsmyndigheten.se/publikationer/rapporter/avfall--transport--fysiskt-skydd/2017/201715/ (last access: 24 October 2023), 2017.
Pensado, O. and Stothoff, S.: Review Assignment for the Swedish Radiation Safety Authority: Independent Canister Integrity Modelling and Dose Assessment, in: SSM Technical Note 2019:22, Swedish Radiation Safety Authority, 2019.
Quintessa: AMBER 5.7 QE-AMBER-2 Version 5.7, Quintessa Henley-on-Thames, UK, https://www.quintessa.org/software/AMBER (last access: 26 October 2023), 2014.
SKB: Long-term safety for the final repository for spent nuclear fuel at Forsmark, Main report of the SR-Site project, Vol. I–III, SKB TR-11-01, Swedish Nuclear Fuel and Waste Management Co, 2011.
SKB: Supplementary information on canister integrity issues, SKB TR-19-15, Svensk Kärnbränslehantering AB, 2019.
SKB: Corrosion of copper after 20 years exposure in the bentonite field tests LOT S2 and A3, Svensk Kärnbränslehantering AB, SKB TR-20-14, 2020.
SSM: The Swedish Radiation Safety Authority's Regulations Concerning the Protection of Human Health and the Environment in Connection with the Final Management of Spent Nuclear Fuel and Nuclear Waste, SSMFS 2008:37, Strålsäkerhetsmyndigheten, 2008.
SSM: Strålsäkerhet efter slutförvarets förslutning. Beredning inför regeringens prövning, Slutförvaring av använt kärnbränsle, SSM Rapport 2018:07, Strålsäkerhetsmyndigheten, 2018 (in Swedish).
SSM: SSM:s granskning av SKB:s komplettering till regeringen om kapselintegritet, Granskningsrapport, dokumentnummer SSM2019-3168-9 07, Strålsäkerhetsmyndigheten, 2019 (in Swedish).
SSM: Strålsäkerhetsmyndighetens granskning av SKB:s analys och rapportering av kopparkorrosionsresultat från försökspaketen A3 och S2 i LOT-projektet vid Äspölaboratoriet, Granskningsrapport, dokumentnummer SSM2021-1539-1 07, Strålsäkerhetsmyndigheten, 2021a (in Swedish).
SSM: Remissvar Ärenden om tillåtlighet enligt miljöbalken och tillstånd enligt lagen om kärnteknisk verksamhet till anläggningar i ett sammanhängande system för slutförvaring av använt kärnbränsle och kärnavfall, dokumentnummer SSM2021-6205-2, 07, Strålsäkerhetsmyndigheten, 2021b (in Swedish).
Stothoff, S. and Manepally, C.: Review and assessment of aspects of the Qeq concept – Main Review Phase, Swedish Radiation Safety Authority, SSM Technical Note 2013:36, 2013.
Szakalos, P. and Leygraf, C.: Review Assignment for the Swedish Radiation Safety Authority: Corrosion of Copper Canister, in: SSM Technical Note 2019:22, Swedish Radiation Safety Authority, 2019.
Szakálos, P., Hultquist, G., and Wikmark, G.: Corrosion of Copper by Water, Electrochemical and Solid-State Letters, 10, C63–C67, 2007.
- Abstract
- Introduction
- Method
- Mechanistic understanding of canister processes
- Repository environment conditions related to canister performance
- Importance analysis based on calculation cases and supporting arguments
- Discussion
- Conclusions
- Code availability
- Data availability
- Author contributions
- Competing interests
- Disclaimer
- Special issue statement
- Acknowledgements
- Review statement
- References
- Abstract
- Introduction
- Method
- Mechanistic understanding of canister processes
- Repository environment conditions related to canister performance
- Importance analysis based on calculation cases and supporting arguments
- Discussion
- Conclusions
- Code availability
- Data availability
- Author contributions
- Competing interests
- Disclaimer
- Special issue statement
- Acknowledgements
- Review statement
- References