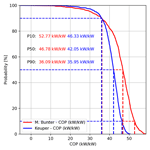
Spatial distribution of Mesozoic deposits and their temperature ranges within the Weser-Wiehengebirge Syncline of the inverted Lower Saxony Basin, Minden area, Germany
Alexander Jüstel
Olga Knaub
Frank Strozyk
Gregor Bussmann
Florian Wellmann
Peter Kukla
The provision of climate-neutral, sustainable, and independent heat sources is an essential part of the ongoing transformation of heating systems in Germany. The city of Minden, located at the junction of the river Weser and the Middleland Canal, with its strong industrial sector, faces a massive transition of how heat and energy will be provided for industrial processes as well as heating in the commercial and residential sectors. In our study, we evaluate the structural requirements for the exploitation and utilization of deep geothermal energy from regional Mesozoic rocks, which are known to source thermal springs in the greater Minden area, and geothermal projects in other parts of the North German Basin. The compilation of geological data, seismic data, and rock properties from wells is used to construct a regional structural model as well as temperature distributions based on depth uncertainties of the respective stratigraphic units. Our investigations indicate several stratigraphic units ranging from the Middle Jurassic, Keuper, and Muschelkalk to the Middle Bunter at depths greater than 4100 m below mean sea level with suitable temperatures greater than 150 ∘C. Seismic data reveal the presence of faults, which may act as a conduit for thermal waters in the northern part of Minden. Our study also provides a basis for further geothermal exploration and exploitation south of Minden, where an operating geothermal system has already been established in the city of Osnabrück and further north, where the potential reservoirs are located at greater depths as shown by hydrocarbon exploration data. First estimations of the geothermal power output for two selected reservoir horizons yield up to 11.3 and 14.3 MW (10 % probability to yield these or higher values), respectively. We conclude that the subsurface of the inverted part of the Lower Saxony Basin principally fulfills the requirements (formation temperatures) for deep geothermal production not only for residential and commercial use but also for industrial processes. However, future detailed reservoir analyses and thermo-hydraulic investigations on a regional scale require additional exploration work like newly acquired seismic surveys and deep exploration wells.
The provision of climate-neutral, sustainable, and regionally independent sources for industrial and residential heat production is a key to the energy transformation in Germany. One part of the solution for meeting these challenges is extracting heat from subsurface hydrothermal systems. It was proposed that direct use of deep geothermal energy or in combination with large-scale heat pumps is able to compensate partially (up to 25 %) for the total German heat demand. This is complemented with additional heat potential seen for aquifer thermal energy storage systems (ATES) and shallow to medium deep geothermal energy (Bracke et al., 2022; Born et al., 2022).
A practical example where an energy transition becomes necessary is the city of Minden, the northernmost city in the state of North Rhine-Westphalia, Germany (Fig. 1). The availability of waterways (Weser River and Middleland Canal) promoted the settlement of numerous industrial branches and businesses within the harbor area to form an extensive industrial park in the northern part of the city outlining the commercial and industrial heat demand. The city of Minden and the larger region around it are already well-known for balneological springs and springs for mineral waters (Fig. 1). The partially Zechstein-salt influenced saline waters are produced from sandstones dated to the Keuper, Muschelkalk, Upper, and Middle Bunter from depths of up to 1000 m (Michel et al., 1998). Future joint utilization of the hydrothermal system requires extensive knowledge about the hydraulic and chemical interactions of the water in the subsurface.
Previous studies in the area around Minden have investigated the subsurface for geothermal energy and other applications. A shallow geothermal project is known from the area of Osnabrück (Fig. 1) where 20 ∘C warm brine is produced from Muschelkalk carbonates in 360 m depth utilized for a public swimming pool (Michalzik et al., 2012). A deep geothermal project in the wider region of Minden is the GeneSys project with its two major parts “GeneSys Horstberg” (Middle Bunter at depths greater 3600 m and formation temperatures of approximately 150 ∘C; e.g., Kehrer et al., 2007; Tischner et al., 2010) and “GeneSys Hannover” (Middle Bunter at depths greater 3400 m and formation temperatures of approximately 170 ∘C; Fig. 1; e.g., Tischner et al., 2010; Hesshaus et al., 2013; Nitschke et al., 2017) initiated by the Federal Institute for Geosciences and Natural Resources (BGR). Other studies in the area of Minden investigated the feasibility of storing CO2 at depths of 3000 m within the Middle Bunter sandstones (Beni et al., 2012).
In this case study, we evaluate the structural requirements for deep geothermal energy production from regional Mesozoic rocks in the subsurface of the city of Minden. Existing information about the structures of the subsurface (Baldschuhn et al., 2001), currently excluded from the TUNB 3D-model of the North German Basin (BGR, 2022), are integrated with the seismic interpretation of a nearby 2D seismic line (RHBGOSTO U940999) and borehole information from hydrocarbon wells provided by the State Office for Mining, Energy and Geology (LBEG) in Hanover. An analytically derived temperature model provides the first ideas about temperature ranges for the different stratigraphic units. The DoubletCalc software (TNO, 2021) is used to calculate an estimate of the geothermal potential (flow rates, thermal outputs) for selected reservoir horizons.
2.1 Geological setting and Mesozoic reservoirs
The city of Minden is located in the southernmost part of the inverted Lower Saxony Basin (Fig. 1; Betz et al., 1987; Voigt et al., 2021), a sub-basin of the Central European Basin (Littke et al., 2008). The Weser- and Wiehengebirge Monocline, located to the south of Minden, exposes sub-horizontally, slightly folded Mesozoic and Permian rocks continuing southwest to the edge of the Münsterland Basin (Fig. 2; Deutloff et al., 1982; Geologischer Dienst Nordrhein-Westfalen, 2003). The area between the Weser-Wiehengebirge Monocline is termed Osning Block (Drozdzewski and Dölling, 2018) and covers a strongly faulted and folded Paleozoic basement consisting of Carboniferous and Devonian strata including Lower Carboniferous and Upper/Middle Devonian limestones acting as primary targets for geothermal exploration in most of the remaining part of North Rhine-Westphalia (Arndt, 2021; Balcewicz et al., 2021). The Weser- and Wiehengebirge Syncline follows the monocline to the north before transitioning into the Quetzen Anticline and Schaumburg-Lippe Syncline before faulting within the Rehburg Graben occurs (Fig. 2; Deutloff et al., 1982; Geologischer Dienst Nordrhein-Westfalen, 2003; Drozdzewski and Dölling, 2018). Major faulting in the area of the Weser- and Wiehengebirge Syncline is not evident (Baldschuhn et al., 2001). The river Weser separates the Wesergebirge in the southeast from the Wiehengebirge and their characteristic Kaiser-Wilhelm monument in the northwest.
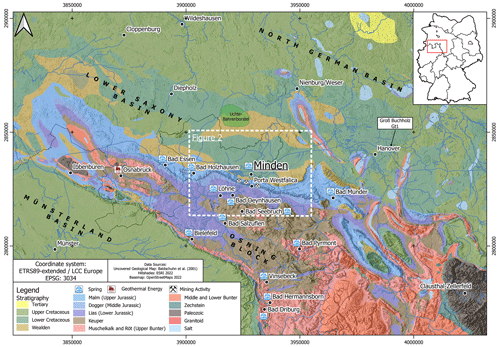
Figure 1Uncovered geological overview map of the southern margin of the Central European Basin (Baldschuhn et al., 2001; Littke et al., 2008). Springs in several cities on the Osning Block are indicated (Michel et al., 1998). Mining locations in the city of Minden are indicated (Deutloff et al., 1982).
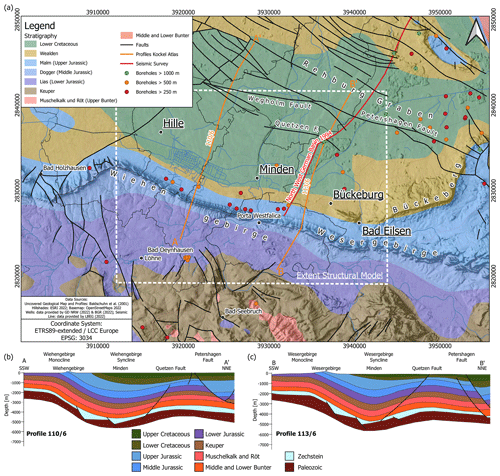
Figure 2(a) Uncovered geological map of the area of Minden zoomed in from Fig. 1 (Baldschuhn et al., 2001). The locations of the cross-section in (b) and (c) and the integrated seismic section (RHBGOSTO U940999) are indicated. Wells with a depth greater than 250 m are shown. (b) & (c) Profiles 110/6 and 113/6 across the Wiehengebirge and Wesergebirge Monocline and Syncline (Baldschuhn et al., 2001).
The stratigraphic column in the area of the city of Minden consists, apart from Quaternary deposits, of Lower Cretaceous rocks exposed within the city limits, Jurassic rocks cropping out along the aforementioned mountain chains and Triassic rocks (Fig. 2; Deutloff et al., 1982). This is equivalent to the North German Basin, another sub-basin of the Central European Basin System (Littke et al., 2008), where several Mesozoic sandstone aquifers have been classified as potential geothermal reservoirs based on their hydraulic properties (porosity/permeability) and thicknesses (Feldrappe et al., 2008; Franz et al., 2018, and references therein). These include the Middle Bunter sandstones of the Solling, Hardegsen, Detfurth, and Volpriehausen formations (e.g., Nitschke et al., 2017; Franz et al., 2018), the Lower to Middle Keuper Stuttgart and Erfurt Formations (e.g., Franz et al., 2015), the Rhaetian sandstones of the Upper Keuper (Franz et al., 2018), Lower and Middle Jurassic sandstones (e.g., Franz et al., 2015, 2018; Kunkel and Agemar, 2019) as well as Lower Cretaceous sandstones (Feldrappe et al., 2008; Franz et al., 2018). Deeper non-hydrocarbon-bearing carbonate reservoirs, such as the Muschelkalk carbonates, have not been a primary exploration target for geothermal energy yet as data is not as abundant as for the hydrocarbon-bearing sandstones. The presence and depth of the Kohlenkalk and Massenkalk carbonates (Arndt, 2021; Balcewicz et al., 2021) remains unknown as data is only available to the Upper Carboniferous in the southern part of the Lower Saxony Basin.
2.2 Geological, regional and structural map data
In addition to the structural maps of the Geotectonic Atlas of Northwest Germany (Baldschuhn et al., 2001), a geological map of Minden (Deutloff et al., 1982) provides valuable input data for the structural modeling of the area between the Weser-Wiehengebirge Monocline and the Quetzen Anticline. The structural maps are available for several stratigraphic units (scale 1:300 000) and are georeferenced. Isolines with a spatial density of 100 m, representing the common depth of a stratigraphic unit at a certain location, were digitized as LineStrings at regular intervals using the QGIS geographic information system (QGIS Development Team, 2022). The structural model was then created based on the digitized data. The recently published TUNB 3D-model of the North German Basin (BGR, 2022), which is also based on the data compiled by Baldschuhn et al. (2001), seismic data sets and well data, does not cover the area of Minden (https://gst.bgr.de/, last access: 10 January 2023).
Zonal statistics of the rasterstats Python package (Perry, 2021) considering the city limits of Minden were used to derive the minimum (edge of the syncline), median and maximum (center of the syncline) depths z of the geological structures for subsequent temperature calculations. An uncertainty for the minimum, median, and maximum depths of each stratigraphic unit is derived from an estimated depth-dependent standard deviation of a normal distribution according to Eq. (1). This uncertainty is defined as there are no deeper wells or depth-converted seismic data available for this study in the immediate vicinity of Minden. Rabbel et al. (2017) also report depth uncertainties with respect to a seismic velocity model misplacing the target horizons by up to 300 m where target depths are lower than in our case. It is therefore assumed that there is a depth uncertainty % for the first 1000 m of the resulting structural model which increases linearly with depth by 1 % per 1000 m. At a depth of 2000 m, the depth uncertainty would be ±11 %, at 3000 m it would be ±12 % and so on. This depth uncertainty is seen as plausible as recent drilling results for geothermal wells in the North German Basin (Hamburg-Wilhelmsburg) encountered such deviations from the original well prognosis (reported at the 13. Norddeutsche Geothermietagung – 12 May 2022; Sävecke, 2022).
Random values of minimum, median and maximum depths were drawn from the normal distribution with the associated depth distribution. Geothermal gradients drawn from a second normal distribution were then multiplied with the randomly drawn depths to obtain a final temperature distribution T in the subsurface for each stratigraphic unit according to Eq. (2). The average geothermal gradient of the Minden area was set to = 34.1 ∘C km−1 with a standard deviation of 2.5 ∘C according to data from GeotIS (Agemar et al., 2012). P10, P50 (median), P90 temperatures in addition to Q1, Q3, minimum and maximum temperatures for boxplots will be reported to describe the temperature ranges.
2.3 Borehole data
Borehole data are available for North Rhine-Westphalia (NRW) and Lower Saxony provided by the Geological Survey NRW (GD NRW) and the LBEG, respectively. Lithostratigraphic logs are mostly available for boreholes in NRW, while geophysical logs from the nearby Uchte and Bahrenborstel gas fields were used for comparison and further investigations (Fig. 3). Only two of the twelve provided borehole data sets including logs were usable. The depths of displayed boreholes range from 250 m to more than 3500 m (Fig. 2). The logs and their data were split according to the reported stratigraphic classifications. Average values from these data ranges were now taken for further investigations.
2.4 2D seismic data
The 2D profile RHBGOSTO U940999 of the “North-West German Basin 1994” survey (Fig. 2) acquired by what is today the ExxonMobil Production Deutschland GmbH was provided by the LBEG in the time domain. A structural and stratigraphic interpretation was performed along the line covering the synclinal structure of Minden and partly the Quetzen Anticline. A seismo-stratigraphic framework was developed after Ahlrichs et al. (2020) compiling lithologies and seismic facies including amplitude, frequency, continuity, bounding relationships, reflector characteristics, and a seismic section example. The framework consists of seismic units representing a mappable interval of seismic reflectors (Ahlrichs et al., 2020). Boundaries of seismic units are identified and classified by marker reflections, unconformities, or correlative conformities (Ahlrichs et al., 2020). A total of eight seismic units (H1 to H8) excluding the basement were defined. A regional velocity model is not available to perform a time-depth conversion. Well markers from deeper wells nearby (e.g. Quetzen 1, drilled 1955, 1077 m deep) could not be converted to a well-to-seismic-tie due to imaging quality around the well and lack of a well path and since no check shots or vertical seismic profilings are available. The depths of tops of seismic units were hence expressed in two-way-travel time (TWT) and converted into thicknesses of seismic units using average velocities from the received borehole data. The depths resulting from stacked unit thicknesses were compared to those of the Geotectonic Atlas (Baldschuhn et al., 2001).
2.5 Geothermal Potential Calculations using DoubletCalc
The geothermal potential expressed as possible flow rates (m3 h−1), coefficient of performance (COP, kW kW−1), and thermal power outputs (MW) is calculated using the DoubletCalc software developed and maintained by TNO (2021). Aquifer properties, doublet and pump properties, and well properties can be defined to estimate the geothermal power of a geothermal doublet. The software uses a Monte-Carlo approach based on provided min-median-max ranges of the aquifer properties and a depth uncertainty of the reservoir of ±10 % (TNO, 2021). DoubletCalc is applied to the Schilfsandstein (Keuper) and the Detfurth Sandstone (Middle Bunter). The parametrization is provided in Table 1.
Franz et al. (2015)Putnis and Mauthe (2001)May et al. (2004)Tischner and Hesshaus (2015)Franz et al. (2015)May et al. (2004)Franz et al. (2018)Agemar et al. (2014)3.1 Structural model and temperature at depth
The constructed structural geological model indicates the depths of the potential reservoir units within the Weser-Wiehengebirge Syncline in the area of Minden. Seven stratigraphic boundaries ranging from the base of the Lower Cretaceous to the base of the Bunter were constructed. The depth of the modeled units, including the potential geothermal reservoirs, vary from 250 ± 23 m TVDSS (true vertical depth sub-sea) for the Base Lower Cretaceous – Top Wealden/Top Upper Jurassic at the edge of syncline to 4981 ± 696 m TVDSS for the Base Lower Bunter – Top Zechstein in the center of the syncline (Table 2). The structural model also depicts the Weser-Wiehengebirge Syncline with the highest depths for the Top Middle Bunter and the overlying stratigraphic units in a stretch from the village of Hille in the west through the city center of Minden and to the northern part of the city of Bückeburg and Bad Eilsen in the east (Fig. 4). This corresponds to a 30 km long NWW-SEE trending structure with depths greater 3000 m. To the south, the model becomes shallower with depths for the Top Middle Bunter of around 800 m TVDSS (Fig. 4). The northern part of the model including the Quetzen Anticline reaches depths of less than 2700 m TVDSS (Fig. 4). Larger faults and associated offsets of approximately up to 100 m are only indicated for the area of Bad Oeynhausen. One fault at the northern margin of the syncline, here termed Quetzen Fault, indicates an offset of 10s of meters (Fig. 4).
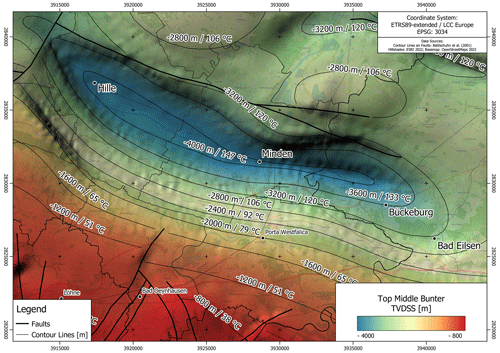
Figure 4Structural model for the top of the Middle Bunter. The structure of the Weser-Wiehengebirge Syncline is clearly visible. Depths of isolines and associated temperatures according to the average geothermal gradient of ∘C km−1 (Agemar et al., 2014).
The corresponding analytically calculated median temperatures for the Top Middle Bunter, the stratigraphic unit holding the deepest reservoir units, range between 85 ∘C for the shallowest depth (2143 ± 239 m) in the marginal area and 151 ∘C for the greatest depth (4103 ± 538 m) for the center of the syncline (Fig. 4). The P90 temperatures for all stratigraphic units range between 19 and 148 ∘C while the P50 temperatures range between 20 and 181 ∘C and the P10 temperatures range between 22 and 216 ∘C (Table 2 and Fig. 5). The values indicate an increase in temperature with depth but also a larger variation of temperatures due to the increase of uncertainty with depth (Fig. 5).
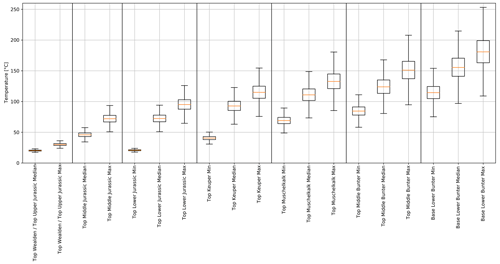
Figure 5Boxplots showing the temperature distributions for the different tops and base (Lower Bunter) of the different stratigraphic units. Minimum, where available, median and maximum temperature scenarios are given for each stratigraphic unit. The temperature ranges are a function of the depth uncertainty of each scenario.
Table 2Depths and temperature ranges for the tops and the base (Lower Bunter) of the different stratigraphic units of the structural model. P10, P50, and P90 as well as temperatures of Fig. 5 are shown (TVDSS = true vertical depth sub-sea). NA = invalid temperatures below the surface temperature of 10.4 ∘C.
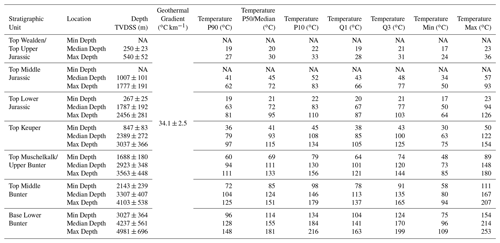
3.2 Seismic interpretation and borehole data
Interpreting horizons H1 to H8 (from bottom to top) on seismic line RHBGOSTO U940999 was enabled based on the strong impedance contrasts in the subsurface (Fig. 6 and Tables 3, 4 and 5). Some of the stratigraphic boundaries are indicated by truncations and onlaps. Due to the absence of well-ties in the immediate vicinity of Minden, the depth conversion approximated by using the mean values of P-velocities from the well log of Uchte T9 (4500 m s−1) serves as a comparison to the structural geological model (Baldschuhn et al., 2001).
Table 3 outlines the maximum depths of the respective stratigraphic boundaries in milliseconds and converted to depth as well as the thickness of the stratigraphic units in milliseconds and meters for the southwestern and northeastern margin of the syncline. Tables 4 and 5 provide a seismo-stratigraphic concept for this study after Ahlrichs et al. (2020) summarizing the nomenclature for seismic reflectors, the respective seismic units, the lithology according to Deutloff et al. (1982), the characteristics for amplitude, frequency, continuity, bounding relationships and further seismic characteristics including a sample seismic section.
Table 3Compilation of results of the seismic interpretation. The table shows the depth and thicknesses of the different stratigraphic units in milliseconds and converted to meters.
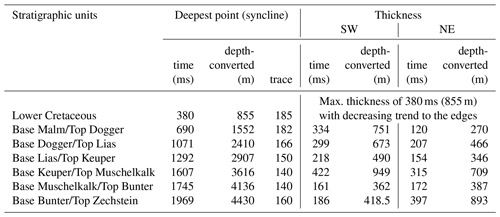
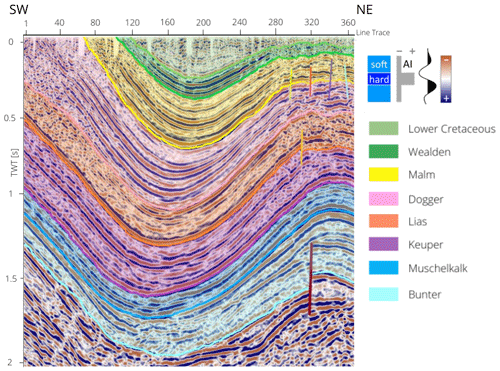
Figure 6Interpreted seismic section RHBGOSTO U940999 displaying the Mesozoic stratigraphic units of the Weser-Wiehengebirge-Syncline (SW) and adjacent Quetzen Anticline (NE).
Table 5Continuation of Table 4. Seismo-stratigraphic concept for this study after Ahlrichs et al. (2020). The table summarizes the nomenclature for seismic reflectors, the respective seismic units, the lithology according to Deutloff et al. (1982), the characteristics for amplitude, frequency, continuity, bounding relationships and further seismic characteristics including a sample seismic section. The size of individual series do not scale to the true timespan.
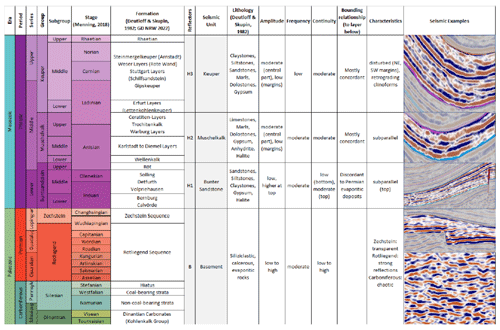
3.3 Geothermal Potential
The stochastic simulations using DoubletCalc resulted in probability distributions (P10, P50, P90) for the expected pump volume flow, the coefficient of performance, and the geothermal power (Fig. 7). The calculated pump volume flows range from 30.8 m3 h−1 (P90 Middle Bunter) to 194.2 m3 h−1 (P10 Keuper). The pump volume flow is generally higher for the Keuper sandstone compared to the Middle Bunter sandstone since permeabilities are lower in the deeper reservoir. The COP ranges from 36.0 kW kW−1 (P90 Keuper) to 52.8 kW kW−1 (P10 Middle Bunter). The resulting geothermal power ranges from 1.8 MW (P90 Middle Bunter) to 14.3 MW (P10 Keuper).
4.1 Analytically derived depths and temperatures
The results of the determined minimum, median and maximum depths as well as associated analytically calculated temperatures derived from a geothermal gradient of 34.1 ± 2.5 ∘C provide a first model-driven temperature estimation of the subsurface and in particular for the stratigraphic units hosting the potential geothermal reservoirs (Table 2; Agemar et al., 2012). At the time of its creation during the late 20th century, the authors of the Geotectonic Atlas integrated all data (seismic data, well data, surface information) and knowledge available to the federal authorities at that time (Baldschuhn et al., 2001). Regional velocity models, check-shots, and well-ties were used to convert acquired seismic two-way-travel-time data to depth. These efforts resulted in a comprehensive atlas outlining the structures in the center of the North German Basin but also at its margins where geophysical data or well data is not as abundant as in the areas of former or current hydrocarbon exploration. The median temperature and depths for the respective stratigraphic units (Table 2) indicate that Cretaceous to Middle Jurassic units, if present in a porous and permeable facies, may only be used for medium-deep geothermal applications with depths of approximately 1000 m and temperatures of 45 ∘C. Reservoirs of the Keuper, Muschelkalk, if present in a porous and permeable facies, excluding the Upper Bunter reach median temperatures of up to 111 ∘C and median depths of approximately 3000 m and may therefore be used directly or in combination with heat pumps for domestic and commercial purposes. Median temperatures of approximately 125 ∘C for the Middle Bunter may not only be suitable for domestic and commercial purposes but also for industrial applications. However, P90 temperatures of 72 ∘C (min depth), median temperatures of 124 ∘C (median depth) and P10 temperatures of 179 ∘C (max depth) outline the current uncertainties associated with the depth and temperature of the reservoirs and stress the need for tighter integration of newly acquired data and petrophysical data to reduce these ranges for a better subsurface temperature prediction.
The stochastic simulations for the geothermal potential of the Keuper and Middle Bunter reservoirs show a range of expected flow rates, COPs, and geothermal power erstimates. The higher temperature in the Middle Bunter reservoir cannot compensate for the higher flow rates for the Keuper reservoir. Therefore, the geothermal power is higher for the shallower, colder but more permeable Keuper reservoir.
4.2 Geological and geophysical interpretations
The structural and stratigraphic investigations of the 2D seismic profile RHBGOSTO U940999 (Fig. 6 and Tables 4, 5) according to the concept of Ahlrichs et al. (2020) allowed for the interpretation of eight major seismic units within the Weser-Wiehengebirge Syncline and Quetzen Anticline above Permian Zechstein evaporites. Seismic characteristics of these units allowed for a correlation to stratigraphic units (Bunter to Lower Cretaceous from bottom to top) in accordance with the local stratigraphy (Deutloff et al., 1982) and the existing structural model after Baldschuhn et al. (2001). Displacements along faults of several hundreds of meters as described by Baldschuhn et al. (2001) are not evident from seismic interpretations. The available seismic section RHBGOSTO U940999 shows relatively minor fault offsets at shallow depths within a mostly folded but hardly faulted area. This implies that, in contrast to fluid pathways described by Michel et al. (1998) for the thermal springs south of Minden, a fault-related ascend of thermal waters is less likely in the subsurface of Minden due to the lack of larger faults. Production from non-faulted sandstone reservoirs may therefore result in lower production rates.
A simplified depth conversion integrating mean seismic P-wave velocities showed results with large deviations from the horizons mapped by Baldschuhn et al. (2001) ranging for all stratigraphic units between −543 m (Base Lower Cretaceous) and 267 m (Base Lower Bunter, Table 6). A negative difference indicates an overestimation of the seismic velocities whereas a positive difference an underestimation of the seismic velocities. This is evident from well logs (Fig. 3) showing seismic velocities of approximately 2500 m s−1 for the Lower Cretaceous and Wealden increasing to approximately 3250 m s−1 for the Jurassic before reaching seismic velocities of 4500 m s−1 and higher in Triassic units. A better understanding of the seismic velocities in the subsurface of Minden is hence necessary to properly position the stratigraphic units at depth.
Therefore, a depth uncertainty between 10 % and 15 % between the structural model and the seismic data is seen as reasonable for the sparse distribution of subsurface data. These uncertainties in depth are of the same magnitude as encountered in recently drilled geothermal wells in the North German Basin (Hamburg-Wilhelmsburg, variations in depths between the original well prognosis and the actual drilling results, reported at the 13. Norddeutsche Geothermietagung – 12 May 2022; Sävecke, 2022).
Table 6Comparison between the depth converted stratigraphic boundaries of seismic line RHBGOSTO U940999 and the constructed structural geological model (TVDSS = true vertical depth sub-sea). A negative difference indicates an overestimation of the seismic velocities whereas a positive difference indicates an underestimation of the seismic velocities.
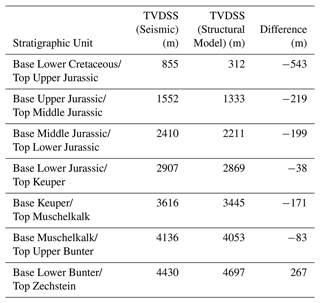
The key results of this study indicate that potential reservoirs or at least their associated stratigraphic intervals are mapped in the subsurface of Minden using the provided 2D seismic data. This reduces the risk for the regions further to the southwest such as Bad Oeynhausen or Bielefeld where stratigraphic units down to the Bunter or even Carboniferous have been intersected by wells before as a continuation of the layers in the subsurface can be assumed. The seismic survey may further act as a starting point for exploration campaigns to come.
However, the true depths and thus formation temperatures of the reservoirs, their thicknesses of the net aquifers, internal structures, diagenetic history, and internal facies distributions remain uncertain. Hence, an exploration well in the Minden area would not only reduce the uncertainty and de-risk the prospect with respect to the presence, depth and temperature of the reservoir rocks but also the uncertainty associated with facies distributions, diagenetic history and petrophysical properties. These new results could then be compared to the existing wells in the Uchte/Bahrenborstel area or the area south of Minden. All obtained results could be integrated into a more comprehensive numerical reservoir model to determine possible flow rates thermal outputs of the respective reservoirs. The consideration of seismic data and structural data promotes the exploration for geothermal energy not only below the city of Minden but also along the entire 30 km long NWW-SEE trending Weser-Wiehengebirge Syncline. With depths greater than 3000 m also in the area of Hille to the west and Bückeberg to the east, the Mesozoic stratigraphic units could also be investigated for their geothermal potential in these regions.
In this study, we have compiled surface and subsurface data from the area of Minden in the inverted part of the Lower Saxony Basin, northwestern Germany. The aim of our study was to create a structural geological model of the subsurface, to interpret a local seismic survey to identify seismic units and stratigraphic units which include siliciclastic and calcareous reservoir units already known and utilized in the larger North German Basin area and to calculate a first temperature distribution integrating associated depth uncertainties of the structural model. The first estimation of flow rates, coefficient of power, and thermal power outputs completes a first investigation of the geothermal potential.
The key results of our investigation indicate the following:
-
The presence of several stratigraphic units within the inverted Lower Saxony Basin in the city of Minden as already postulated by Baldschuhn et al. (2001) ranging from the Upper Jurassic, Keuper, Muschelkalk and Middle Bunter down to depths greater 4100 m TVDSS that may contain geothermal reservoir units such as sandstones but also carbonates is confirmed by interpreting a previously unpublished 2D seismic line.
-
The stratigraphic units hosting potential geothermal reservoirs could be identified on seismic line RHBGOSTO U940999 and thus their presence could be proven in the immediate vicinity of Minden using a seismo-stratigraphic concept.
-
The seismic line and the constructed structural geological model further outline the structure of the Weser-Wiehengebirge Syncline, a more than 30 km long NWW-SEE trending structure with depths greater than 3000 m and minor faulting at its edges which may still propagate to the central and hence deeper parts of the syncline to promote preferential fluid pathways and thus be suitable for the exploration for geothermal energy.
-
The analytically calculated temperatures in the subsurface reach values above 150 ∘C for the deepest reservoir sections and are thus suitable to extract heat from the subsurface to supply the residential and commercial sector directly or with the use of heat pumps and to provide heat for industrial processes.
-
The uncertainties associated with the structural geological model ranging between 23 m (Base Lower Cretaceous) and 696 m (Base Lower Bunter) and temperature distributions (e.g. 85 to 151 ∘C, median temperatures of the Middle Bunter) outline the necessity of exploration activities to reduce those uncertainties to decide on a utilization concept for the thermal power that can be produced in the end.
-
Stochastic simulations of two potential geothermal reservoirs show that the shallower and therefore cooler but more permeable Keuper reservoir results in geothermal power outputs between 2.3 MW (P90) and 14.3 MW (P10) compared to 1.8 to 11.3 MW in the Bunter.
Our results indicate multiple potential geothermal reservoirs at different depth intervals in the subsurface of Minden and provide the structural geological basis for further numerical modeling applications and exploration activities in the future. The calculation of the geothermal potential for a single doublet system yields bandwidths of geothermal power outputs for the different reservoirs. The number of doublets may be scalable along the mapped structure to develop a geothermal system in the subsurface of Minden and surrounding areas to provide geothermal energy for the domestic, commercial and/or industrial sectors.
GIS data, Excel Files, and Jupyter Notebooks containing the Python codes used to create figures and to perform calculations can be downloaded from https://doi.org/10.5281/zenodo.7488833 (Jüstel et al., 2022). The seismic data and borehole data such as geophysical logs and well reporting may not be published due to license agreements but can be requested from LBEG or GD NRW for further scientific or commercial purposes.
GB was in charge of the project administration. AJ and OK conceptualized the study, requested, compiled and evaluated the available data. FW, PK, and FS supervised the thesis of OK from a scientific point of view. The thesis of OK was further associated with numerical simulations in this area. AJ and OK prepared the original draft with significant contributions in form of reviews and edits from all co-authors. GB, FW, and PK funded AJ and OK through their departments at Fh IEG.
The contact author has declared that none of the authors has any competing interests.
Publisher’s note: Copernicus Publications remains neutral with regard to jurisdictional claims in published maps and institutional affiliations.
This article is part of the special issue “European Geosciences Union General Assembly 2022, EGU Division Energy, Resources & Environment (ERE)”. It is a result of the EGU General Assembly 2022, Vienna, Austria, 23–27 May 2022.
The authors would like to thank the European Geoscience Union for providing the opportunity to contribute to the ERE special issue 2022 in Advances in Geosciences (ADGEO). Further, the authors would like to thank the Landesamt für Bergbau, Energie und Geologie (LBEG) in Hanover and the Geological Survey of NRW (GD NRW) in Krefeld for providing the seismic and borehole data for this study. Seismic interpretation was performed using the IHS Kingdom Suite using a commercial license.
The main authors, AJ and OK, received funding as part of their employment as scientific assistant (WiMi)/PhD candidate and research assistant (WiHi)/master student at Fraunhofer IEG, respectively.
This open-access publication was funded by the RWTH Aachen University.
This paper was edited by Michael Kühn and reviewed by Thomas Baumann and one anonymous referee.
Agemar, T., Schellschmidt, R., and Schulz, R.: Subsurface temperature distribution in Germany, Geothermics, 44, 65–77, https://doi.org/10.1016/j.geothermics.2012.07.002, 2012. a, b
Agemar, T., Weber, J., and Schulz, R.: Deep Geothermal Energy Production in Germany, Energies, 7, 4397–4416, https://doi.org/10.3390/en7074397, 2014. a, b
Ahlrichs, N., Hübscher, C., Noack, V., Schnabel, M., Damm, V., and Krawczyk, C.: Structural Evolution at the Northeast North German Basin Margin: From Initial Triassic Salt Movement to Late Cretaceous-Cenozoic Remobilization, Tectonics, 39, e2019TC005927, https://doi.org/10.1029/2019TC005927, 2020. a, b, c, d, e, f
Arndt, M.: 3D modelling of the Lower Carboniferous (Dinantian) as an indicator for the deep geothermal potential in North Rhine-Westphalia (NRW, Germany), Z. Dtsch. Geol. Gesell., 172, 307–324, https://doi.org/10.1127/zdgg/2021/0279, 2021. a, b
Balcewicz, M., Ahrens, B., Lippert, K., and Saenger, E. H.: Characterization of discontinuities in potential reservoir rocks for geothermal applications in the Rhine-Ruhr metropolitan area (Germany), Solid Earth, 12, 35–58, https://doi.org/10.5194/se-12-35-2021, 2021. a, b
Baldschuhn, R., Binot, F., Fleig, S., and Kockel, F. (Eds.): Geotektonischer Atlas von Nordwest-Deutschland und dem deutschen Nordsee-Sektor, Schweizerbart Science Publishers, Stuttgart, Germany, http://www.schweizerbart.de//publications/detail/isbn/9783510958818/Geologisches_Jahrbuch_Reihe_A_Heft_1 (last access: 10 January 2023), 2001. a, b, c, d, e, f, g, h, i, j, k, l, m, n
Beni, A., Kühn, M., Meyer, R., and Clauser, C.: Numerical Modeling of a Potential Geological CO 2 Sequestration Site at Minden (Germany), Environ. Model. Assess., 17, 337–351, https://doi.org/10.1007/s10666-011-9295-x, 2012. a
Betz, D., Führer, F., Greiner, G., and Plein, E.: Evolution of the Lower Saxony Basin – Compressional Intra-Plate Deformations in the Alpine Foreland, Tectonophysics, 137, 127–170, https://doi.org/10.1016/0040-1951(87)90319-2, 1987. a
BGR, LAGB, LBEG, LBGR, LLUR, and LUNG: 3D-Modell des geologischen Untergrundes des Norddeutschen Beckens (Projekt TUNB), Erstveröffentlichung 2021, Version 2022, https://gst.bgr.de, last access: 16 October 2022. a, b
Born, H., Bracke, R., Eicker, T., and Rath, M.: Roadmap Oberflächennahe Geothermie – Erdwärmepumpen für die Energiewende – Potenziale, Hemnisse und Handlungsempfehlungen, Tech. rep., Fraunhofer-Einrichtung für Energieinfrastrukturen und Geothermie IEG, https://doi.org/10.24406/publica-70, 2022. a
Bracke, R., Huenges, E., Acksel, D., Amann, F., Bremer, J., Bruhn, D., Budt, M., Bussmann, G., Gorke, J.-U., Grun, G., Hahn, F., Hanßke, A., Kohl, T., Kolditz, O., Regenspurg, S., Reinsch, T., Rink, K., Sass, I., Schill, E., Schneider, C., Shao, H., Teza, D., Thien, L., Utri, M., und Will, H.: Roadmap Tiefe Geothermie für Deutschland – Handlungsempfehlungen für Politik, Wirtschaft und Wissenschaft für eine erfolgreiche Wärmewende, Tech. rep., Fraunhofer-Einrichtung für Energieinfrastrukturen und Geothermie IEG and Helmholtz-Gemeinschaft, 37 pp., https://doi.org/10.24406/ieg-n-645792, 2022. a
Deutloff, O. and Skupin, K.: Geologische Karte von Nordrhein-Westfalen 1: 100 000 – Blatt C 3918 Minden, Geologisches Landesamt Nordrhein-Westfalen, Krefeld, Germany, https://www.opengeodata.nrw.de/produkte/geologie/geologie/GK/ISGK100/GK100analog/GK100-C3918-Minden_EPSG25832_JPEG.zip (last access: 17 January 2023), 1982.
Deutloff, O., Kühn-Velten, H., Michel, G., Skupin, K., and Hilden, H.: Geologische Karte von Nordrhein-Westfalen 1 : 100 000 – Blatt C3918 Minden, Geologisches Landesamt Nordrhein-Westfalen, Krefeld, 1982. a, b, c, d, e, f, g, h
Drozdzewski, G. and Dölling, M.: Elemente der Osning-Störungszone (NW-Deutschland) – Leitstrukturen einer Blattverschiebungszone, scriptum online – Geowissenschaftliche Arbeitsergebnisse aus Nordrhein-Westfalen, 7, 1–39, 2018. a, b
Feldrappe, H., Obst, K., and Wolfgramm, M.: Die mesozoischen Sandsteinaquifere des Norddeutschen Beckens und ihr Potential für die geothermische Nutzung [Mesozoic sandstone aquifers of the North German Basin and their potential for the geothermal utilization], Z. Geol. Wiss., 36, 199–222, 2008. a, b
Franz, M., Wolfgramm, M., Barth, G., Nowak, K., Zimmermann, J., Budach, I., and Thorwart, K.: Schlussbericht Verbundprojekt: Identifikation hydraulisch geeigneter Bereiche innerhalb der mesozoischen Sandsteinaquifere in Norddeutschland [Final Report R&D project: Hydraulic properties of Mesozoic sandstone aquifers of North Germany], Tech. rep., TU Bergakademie Freiberg, 333 pp., 2015. a, b, c, d
Franz, M., Barth, G., Zimmermann, J., Budach, I., Nowak, K., and Wolfgramm, M.: Geothermal resources of the North German Basin: exploration strategy, development examples and remaining opportunities in Mesozoic hydrothermal reservoirs, Geol. Soc. Spec. Publ., 469, 193–222, https://doi.org/10.1144/SP469.11, 2018. a, b, c, d, e, f
Geologischer Dienst Nordrhein-Westfalen: Geologie im Weser- und Osnabrücker Bergland, Geologischer Dienst Nordrhein-Westfalen, Krefeld, 219 pp., ISBN 978-3860299326, 2003. a, b
Geologischer Dienst Nordrhein-Westfalen: Die Erdgeschichte unseres Landes, https://www.gd.nrw.de/ge_ev_stratigraphie.htm (last access: 17 January 2023), 2022.
Hesshaus, A., Houben, G., and Kringel, R.: Halite clogging in a deep geothermal well – Geochemical and isotopic characterisation of salt origin, Phys. Chem. Earth, Parts A/B/C, 64, 127–139, https://doi.org/10.1016/j.pce.2013.06.002, 2013. a
Jüstel, A., Knaub, O., Strozyk, F., Bussmann, G., Wellmann, F., and Kukla, P.: Spatial distribution of Mesozoic deposits and their temperature ranges within the Weser-Wiehengebirge Syncline of the inverted Lower Saxony Basin, Minden area, Germany [Data set], in: Advances in Geosciences (1.0.0), EGU General Assembly 2022 (EGU 2022), Vienna, Austra, Zenodo [data set], https://doi.org/10.5281/zenodo.7488833, 2022. a
Kehrer, P., Orzol, J., Jung, R., Jatho, R., and Junker, R.: The GeneSys project a contribution of Geozentrum Hannover to the development of Enhanced Geothermal Systems (EGS), Z. Deutsch. Gesell. Geow., 158, 119–132, https://doi.org/10.1127/1860-1804/2007/0158-0119, 2007. a
Kunkel, C. and Agemar, T.: Hydraulic Characterization of Potential Geothermal Reservoirs in the North German Basin, European Geothermal Congress, 2019, 1–8, 2019. a
Littke, R., Bayer, U., Gajewski, D., and Nelskamp, S.: Dynamics of Complex Intracontinental Basins – The Central European Basin System, Springer Berlin, Heidelberg, Berlin, Heidelberg, https://doi.org/10.1007/978-3-540-85085-4, 2008. a, b, c
May, F., Krull, P., and Gerling, P.: CO2 Storage Scenarios in North Germany – GESTCO Project Case Studies, Tech. rep., Bundesanstalt für Geowissenschaften und Rohstoffe, https://www.bgr.bund.de/EN/Themen/Nutzung_tieferer_Untergrund_CO2Speicherung/Projekte/CO2Speicherung/Abgeschlossen/Nur-Deutsch/Gestco/GESTCO_project_case_studies_2004.pdf? (last access: 10 January 2023), 2004. a, b
Menning, M.: Die Stratigraphische Tabelle von Deutschland 2016 (STD 2016) [The Stratigraphic Table of Germany 2016 (STG 2016)], Z. Dtsch. Geol. Gesell., 169, 105–128, https://doi.org/10.1127/zdgg/2018/0161, 2018.
Michalzik, D., Fromme, K., Steffahn, J., Achilles, H.-H., and Schockemöhle, C.: Das Geothermieprojekt der Stadtwerke Osnbrück, Schriftenreihe der Deutschen Gesellschaft für Geowissenschaften, 8 – GeoHannover, 216, ISBN 978-3-510-49228-2, 2012. a
Michel, G., Adams, U., and Schollmayer, G.: Mineral- und Heilwässervorkommen in Nordrhein-Westfalen, Geologisches Landesamt Nordrhein-Westfalen, Krefeld, ISBN 978-3-86029-930-2, 80 pp., 1998. a, b, c
Nitschke, F., Held, S., Himmelsbach, T., and Kohl, T.: THC simulation of halite scaling in deep geothermal single well production, Geothermics, 65, 234–243, https://doi.org/10.1016/j.geothermics.2016.09.009, 2017. a, b
Perry, M.: python-rasterstats, https://github.com/perrygeo/python-rasterstats (last access: 10 January 2023), 2021. a
Putnis, A. and Mauthe, G.: The effect of pore size on cementation in porous rocks, Geofluids, 1, 37–41, https://doi.org/10.1046/j.1468-8123.2001.11001.x, 2001. a
QGIS Development Team: QGIS Geographic Information System, QGIS Association, https://www.qgis.org (last access: 10 January 2023), 2022. a
Rabbel, W., Jusri, T., Köhn, D., Bahadur Motra, H., Niederau, J., Schreiter, L., Thorwart, M., and Wuttke, F.: Seismic Velocity Uncertainties and their Effect on Geothermal Predictions: A Case Study, Energy Procedia, 125, 283–290, https://doi.org/10.1016/j.egypro.2017.08.178, european Geosciences Union General Assembly 2017, EGU Division Energy, Resources & Environment (ERE), 2017. a
Sävecke, T.-T.: Transformation städtischer Wärmeversorgung mit Geothermie – Hamburg Wilhelmsburg, Presented at the 13. Norddeutsche Geothermie Tagung, 12 May, https://norddeutsche-geothermietagung.de/vortraege/2022/02_saevecke_transformation_staedtischer_waermeversorgung.pdf (last access: 10 January 2023), 2022. a, b
Tischner, T. and Hesshaus, A.: Stimulationsmaßnahmen, hydraulische Tests und geochemische Untersuchungen, in: Erdwärmegewinnung mittels Generierter geothermischer Systeme (GeneSys) – Teil 1: Testarbeiten in der Bohrung Horstberg Z1 und Abteufen der Bohrung Groß Buchholz Gt1, edited by: Gerling, J., Tischner, T., Kosinowski, M., and Bräuer, V., Chap. 3, 35–90, Schweizbart Science Publishers, Stuttgart, Germany, ISBN 978-3-510-96853-4, 2015. a
Tischner, T., Evers, H., Hauswirth, H., Jatho, R., Kosinowski, M., and Sulzbacher, H.: New Concepts for Extracting Geothermal Energy from One Well: The GeneSys-Project, Proceedings World Geothermal Congress 2010, 2010, 1–5, https://www.geothermal-energy.org/pdf/IGAstandard/WGC/2010/2272.pdf (last access: 10 January 2023), 2010. a, b
TNO: DoubletCalc, https://www.nlog.nl/en/tools (last access: 10 January 2023), 2021. a, b, c
Voigt, T., Kley, J., and Voigt, S.: Dawn and dusk of Late Cretaceous basin inversion in central Europe, Solid Earth, 12, 1443–1471, https://doi.org/10.5194/se-12-1443-2021, 2021. a