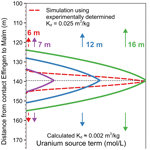
Uranium migration lengths in Opalinus Clay depend on geochemical gradients, radionuclide source term concentration and pore water composition
Theresa Hennig
Michael Kühn
Safety assessments of highly radioactive waste disposal sites are done based on simulation of radionuclide migration lengths through the containment providing rock zone. For a close to real case situation, the present model concept established for uranium is derived from the hydrogeological evolution and geochemical and mineralogical data measured at the deep geothermal borehole Schlattingen including the effect of geo-engineered barriers on the source term. In the Schlattingen area, the Opalinus Clay is tectonically undeformed compared to the Mont Terri anticline and represents the geochemical and temperature conditions at the favoured disposal depth. The geochemical conditions are more or less constant with slightly decreasing concentrations of pore water components towards the footwall aquifer. Uranium migrates less compared to the Opalinus Clay system at Mont Terri, where gradients of pore water geochemistry towards the embedding aquifers are more pronounced. This means, stable geochemical conditions with no or low concentration gradients are to be favoured for a safe disposal since migration lengths strongly depend on spatial and temporal variation of the hydrogeological and geochemical conditions within the host formation. The engineered barriers reduce the source term concentration what, in turn, is associated with a decrease in uranium migration. Stable geochemical conditions further enable the application of the Kd approach to estimate the impact of the barriers. The hydrogeological system must always be considered when quantifying radionuclide migration.
- Article
(2077 KB) - Full-text XML
- BibTeX
- EndNote
Safety assessments of disposal sites for highly radioactive wastes are done based on the quantification of potential radiological impacts on human health and the environment (IAEA, 2012). For this, radionuclide migration through the containment providing rock zone (CRZ), e.g. the rock unit hosting the waste containers and surrounding low permeable formations (> 100 m thickness), needs to be simulated for time periods of up to one million years. Thereby, potential spatial and temporal hydrogeochemical changes within the entire rock zone and their associated impacts on the transport processes as well as the multi-barrier system of common disposal concepts need to be considered (IAEA, 2003). In this context, reactive transport modelling is a powerful tool to cover the required scales and to quantify transport processes as a function of geochemical and mineralogical variations.
The meaningfulness of the migration lengths resulting from reactive transport simulations highly depends on the model concept, used input and thermodynamic data. In the case of uranium in the Opalinus Clay system at Mont Terri (Switzerland), previous numerical studies have shown that the range extends from 5 m using experimentally determined transport parameters in a diffusion model following Fick's laws (Joseph et al., 2013b; Hennig, 2022), over 50 m using process-based approaches and taking hydrogeology into account (Hennig and Kühn, 2021b), up to 80 m depending on the thermodynamic data set used (Hennig and Kühn, 2022). This range emphasises the uncertainty associated with reactive transport simulations and how strongly migration lengths can be over- or underestimated, respectively, which might have direct consequences for the safety of a potential disposal site. However, these results are maximum scenarios because geochemical gradients are present at Mont Terri due to the diffusive exchange between the CRZ and its surrounding aquifers over millions of years and the geo-engineered barriers were not considered. For the disposal of highly radioactive waste, an area that is tectonically less or undeformed compared to the Mont Terri anticline and at a greater depth is favoured (IAEA, 2003). Therefore, the hydrogeological setting at Mont Terri as well as the geochemical and temperature data might not be representative for potential disposal sites. For instance, recent pore water data from the Swiss favoured siting area in “Nördlich Lägern” indicates more or less stable geochemical conditions within the CRZ at a depth of around 850 m (Nagra, 2022). Furthermore, the geo-engineered barriers shall retain the radionuclides within the near-field as long as possible by a minimization of their release from the spent fuel and their migration into the host rock. In the Swiss disposal concept for highly radioactive waste (Nagra, 2021), for instance, the geo-engineered barriers comprise of thick-walled steel containers and bentonite or a bentonite/sand mixture as backfill material for the tunnels. This, in turn, would lead to a decreased source term concentration compared to the one previously used. Consequently, a realistic model concept includes the effect of multiple barriers on the mobility and the hydrogeological setting.
Besides Switzerland, many countries in Europe, such as France, Belgium, Hungary and Germany, have chosen or consider argillaceous formations for the disposal of highly radioactive waste. For instance, the retention behaviour of uranium in the Callovo-Oxfordian Clay (France) has been investigated in batch experiments under various conditions relevant for highly radioactive waste disposal (Montavon et al., 2022). It has been shown that the sorption capacity of the clay formation can vary significantly depending on the investigated geochemical conditions, in particular redox potential, pH, partial pressure of CO2 (pCO2) and calcium concentration. Accordingly, the results obtained in this study might also be relevant for other clay formations with similar pore water geochemistry.
The migration of uranium as main component of spent fuel in the Opalinus Clay, the chosen host rock for the disposal of nuclear wastes in Switzerland, is used as an example for the quantification of radionuclide migration close to a real case situation. Therefore, the conceptual model already applied to simulate the present-day pore water chemistry profile measured at Mont Terri (Hennig and Kühn, 2021b, 2022) is now transferred to the region of the deep geothermal borehole Schlattingen, which is in vicinity to one of the three siting areas in Switzerland (“Zürich Nordost”; Nagra, 2022). At Schlattingen, geochemistry and mineralogy of the Opalinus Clay and surrounding formations have been intensively investigated and supplemented with hydrogeological knowledge from the exploration borehole Benken (Gimmi et al., 2007; Wersin et al., 2016, 2018), which provide a good database for the reactive transport simulations conducted in the present study.
One-dimensional reactive transport simulations are conducted with PHREEQC Version 3 (Parkhurst and Appelo, 2013) using Fick’s laws, surface complexation and cation exchange to quantify diffusion and sorption processes of uranium as a function of pore water geochemistry and mineralogy. In a first step, the present-day pore water chemistry profile measured at Schlattingen is modelled and used in a second step as initial conditions for the subsequent simulation of uranium migration. The thermodynamic data set is based on the PSI/Nagra database version 12/07 (Thoenen et al., 2014) including the thermodynamic data for uranium published by the NEA (Grenthe et al., 2020) as well as a compilation of surface complexation data for the hydroxo-complexes of uranium to quantify sorption on the inherent clay minerals using the bottom-up approach (Marques Fernandes et al., 2015; Stockmann et al., 2017). This surface complexation data set was compiled in the context of an uranium sorption experiment (Joseph et al., 2013a) and has already been applied (Hennig et al., 2020; Hennig and Kühn, 2021a, b). Illite and montmorillonite are the cation exchange phases in the simulations, which are initially equilibrated with the pore water solution (Hennig et al., 2020). Further details on the integration of uranium sorption processes, all surface parameters and reactions as well as cation exchange reactions can be found in Table 1 of the supplement of Hennig et al. (2020). Ionic strengths of the pore waters within the CRZ as well as of the groundwaters do not exceed 0.5 mol L−1 (Table 1), and therefore the Davies approach (Davies and Shedlovsky, 1964) is considered as appropriate to represent ion-ion interactions (Stockmann et al., 2017; Noseck et al., 2018). From the Schlattingen borehole, a temperature of 45 ∘C was measured at a depth of 799–833 m (Wersin et al., 2016) corresponding to the transition from Brown Dogger to Opalinus Clay (Fig. 1). However, scoping calculations have shown that the effect of the higher in-situ temperature at Schlattingen compared to pore water data measured at room temperature is < 5 %, except for alkalinity with a 35 % lower concentration (Wersin et al., 2016). Furthermore, from uranium diffusion experiments for different temperatures (25 and 60 ∘C) it has been concluded that elevated temperatures increase diffusion but also sorption so that both compensate each other (Joseph et al., 2013b). Therefore, all geochemical calculations are conducted for a temperature of 25 ∘C as in line with the database. The applied model constraints have been validated in previous studies.
Geochemical and mineralogical data of the Opalinus Clay at the area of the deep geothermal borehole Schlattingen (Wersin et al., 2016) is used as representative for conditions close to the suitable disposal depth (600–700 m) and tectonically favourable and stable conditions (Nagra, 2002). The borehole is located around 9 km northeast from the deep exploration borehole Benken between the northern part of the Swiss Molasse Basin and the Tabular Jura (Gimmi et al., 2007; Wersin et al., 2016). Here, the sedimentary rocks are nearly horizontally bedded (Gimmi et al., 2007). At Schlattingen, mineralogical and pore water data of a 280 m thick low permeable section has been obtained among others by X-ray diffractometry, aqueous extraction, squeezing tests as well as pCO2 measurements (Wersin et al., 2016). The CRZ consists of the Effingen Member (ca. 25 m), Brown Dogger (ca. 75 m), Opalinus Clay (ca. 120 m), Lias (ca. 50 m) and the upper part of the Keuper (ca. 10 m; considered borehole depth 733–1013 m, Fig. 1). Further informations on the analytical procedures are given in Wersin et al. (2013). For the clay minerals, an averaged composition (43 wt %, Fig. 1) based on measured values (Wersin et al., 2016) is used across the entire modelling domain since pore water geochemistry is more decisive for uranium migration than variations in the clay mineral quantity across a formation, for instance, as present at Mont Terri between the Opalinus Clay litho-facies (Hennig et al., 2020; Hennig and Kühn, 2021b; Hennig, 2022). Pore water data obtained from squeezed rock samples is a good representation of the in-situ conditions (Mazurek et al., 2015; Wersin et al., 2016) and has already been used as comparison in previous simulations of present-day pore water chemical profiles at Schlattingen (Wersin et al., 2018). Modelled profiles of cation and anion concentrations are based on and compared with published geochemical and mineralogical data.
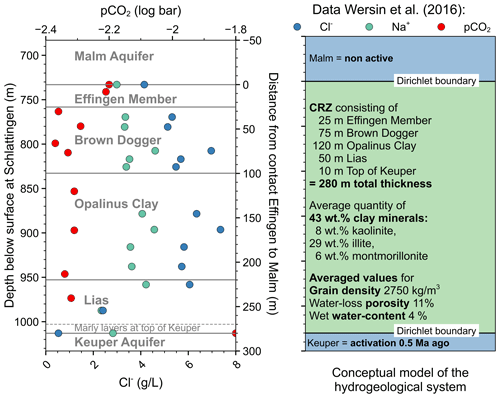
Figure 1The conceptual model consists of the containment providing rock zone (CRZ, green box), which is surrounded by the aquifers in the Malm and Keuper (blue boxes). According to Wersin et al. (2018), the latter was activated around 0.5 Ma ago, whereas the groundwater in the Malm is characterised by stagnant, e.g. no flow, conditions. Present-day pore water chemistry profile is exemplary shown by measured sodium (light blue) and chloride (dark blue) concentrations as well as by the partial pressures of carbon dioxide (pCO2, red; Wersin et al., 2016). Boundary conditions are kept constant (Dirichlet boundary).
The CRZ is surrounded by aquifers in the Malm and Keuper lithologies (Fig. 1). The original marine pore water is affected by water-rock interactions, such as dissolution and precipitation of minerals, as well as by diffusive exchange with the surrounding units of higher permeabilities (Wersin et al., 2016). The latter explains the slight decrease in the concentrations of the pore water components towards the underlying Keuper aquifer (Fig. 1). Concentration profiles of chloride and sodium are exemplarily given for all pore water components. Further, measured pCO2 values are included since this is the governing factor for uranium speciation, and hence sorption (Joseph et al., 2011; Hennig et al., 2020; Hennig and Kühn, 2022). Besides cation exchange reactions with the inherent clay minerals that control the concentrations of the main cations, calcite and pyrite are omnipresent minerals. Both formed during early diagenesis and remain stable during all the burial history and govern the geochemical conditions within the pore water, namely pH and redox conditions, respectively (Pearson et al., 2003, 2011; Lerouge et al., 2014; Wersin et al., 2016, 2018). Celestite is present as cement in some silty lenses and as fills in fractures in Opalinus Clay from Mont Terri (Lerouge et al., 2015; Pekala et al., 2019) and from the Benken borehole (Lerouge et al., 2014). It seems to be present during all the diagenesis process and most likely constrains the sulphate concentration since squeezed waters are close to equilibrium (Wersin et al., 2016, 2022). Therefore, equilibrium with calcite, celestite, pyrite and magnetite as Fe(II)Fe(III) mixed oxide, which is also observed in Opalinus Clay (Aubourg et al., 2021), is applied in our simulations to control pore water geochemistry as a function of pCO2. For the pCO2 a constant value of 10−2.3 bar is chosen because measured values only show minor variations across the CRZ (Fig. 1). The knowledge on the hydrogeological conditions and paleohydrogeological evolution is based on extensive studies from the nearby Benken borehole since the situation at Schlattingen is not well known (Gimmi et al., 2007; Wersin et al., 2018). In the overlying Malm aquifer, the chemistry mirrors a mixture of freshwater and marine components based on data from Benken with fairly stagnant, and thus constant, conditions over the last one million years (Wersin et al., 2018). The underlying Keuper aquifer was likely flushed with meteoric water within the last 0.5–1 Ma (Gimmi et al., 2007), which is also valid for the Schlattingen site (Wersin et al., 2018). Therefore, we choose an activation time of the Keuper aquifer 0.5 Ma ago (Fig. 1). According to Wersin et al. (2018), the composition of the Malm aquifer equals the top sample of the Effingen Member, whereas the Keuper groundwater composition is taken from the Benken borehole. The initial pore water composition in the CRZ prior to the activation of the aquifers is assumed to correspond to the maximum values measured today (Wersin et al., 2016), which are in equilibrium with the mineral assemblage (Table 1). Accordingly, resulting concentrations, as given in Table 1 for CRZ, are initially equal across the modelling domain and represent the starting point for the simulation of the pore water chemistry. That means, the boundary condition at the bottom, i.e. the water composition of the Keuper aquifer, changes to the concentrations given in Table 1 and diffusive exchange between Keuper aquifer and CRZ is modelled for 0.5 Ma. Consequently, the hydrogeological system needs to be taken into account for the assessment of radionuclide migration.
Table 1According to Wersin et al. (2018), the initial composition of the Malm aquifer at the hanging wall boundary equals the uppermost sample in the Effingen Member, whereas the groundwater composition in the Keuper aquifer at the footwall boundary is taken from measurements at the nearby Benken borehole. Values are given for equilibrium with the mineral assemblage for the given pCO2 (indicated by*). CRZ = containment providing rock zone.
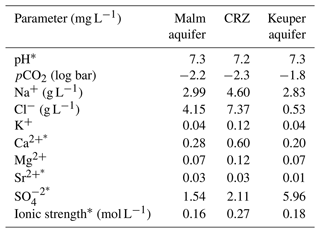
Values for porosity, grain density, water content as well as clay mineral quantities are assumed to be constant throughout the CRZ. The corresponding values are averaged based on the Schlattingen data (Wersin et al., 2016) and are given in Fig. 1. The distribution coefficient Kd (m3 kg−1) is defined as ratio between the species concentration adsorbed on the solid phase and present in the liquid phase. It is calculated as observable from the PHREEQC results and used to outline potential differences of the sorption capacity. Diffusive transport is quantified using Fick’s law. For modelling the present-day profile of the pore water chemistry, the pore diffusion coefficient Dp (m2 s−1) of tritiated water (HTO) at 45 ∘C is used (value 1.77 × 10−10 m2 s−1). This value was calculated by Wersin et al. (2018) from the averaged experimental values, which were measured at room temperature from Schlattingen Opalinus Clay samples, following the correction procedure described by Van Loon et al. (2005). For the subsequent simulation of uranium migration, a Dp of 1.72 × 10−11 m2 s−1 is used as determined by Joseph et al. (2013b) in an uranium diffusion experiment with Opalinus Clay. In the geochemical system of the Opalinus Clay, uranium is mainly present as U(VI) with the ternary complex Ca2UO2(CO3)3 as predominant species (Joseph et al., 2013b). This was verified by time-resolved laser-induced fluorescence spectroscopy in the frame of a batch sorption experiment (Joseph et al., 2011) and is also in line with previous geochemical simulations, which used the same thermodynamic data as in this study (Hennig and Kühn, 2022). Thus, the predominant species is consistent between experiments and modelled system. Since physico-chemical parameters are assumed to be constant, this also applies to the diffusion coefficient.
Engineered barriers, e.g. waste canisters and backfill material, shall avoid radionuclide migration into the host rock, and thus reflect the source term. This is integrated by using a reduced concentration of 10−7 and 10−8 mol L−1 compared to the maximum value of 10−6 mol L−1 (Joseph et al., 2013b; Hennig et al., 2020; Hennig and Kühn, 2021b, 2022). The uranium source term to mimic the failed high-level waste canisters is integrated about in the centre of the model (depth of 140 m from contact Effingen to Malm corresponding to 870 m below surface, Fig. 1) by a mineral equilibrium with uraninite together with pyrite so that uranium can migrate in both directions.
3.1 Modelling of pore water chemistry profile
Present-day profiles of cation and anion concentrations at Schlattingen are modelled based on measured data from the deep geothermal borehole and the knowledge on the hydrogeological conditions and paleohydrogeological evolution gained from studies at the nearby Benken borehole (Gimmi et al., 2007; Wersin et al., 2016, 2018). Simulation results are exemplary shown for the main components of the pore water, sodium and chloride (light and dark blue lines), as well as for the constituents governing uranium speciation (Table 2, Hennig et al., 2020; Hennig and Kühn, 2022): strontium (orange line), magnesium (green line), calcium (red line) and pH (purple line, Fig. 2). Alkalinity (not shown) is more or less constant with concentrations around 120 mg L−1 with higher concentrations towards the adjacent formations (< 240 mg L−1). Modelled pH increases from around 7.2 at the the centre of the CRZ (depth of 870 m below surface or 140 m from contact Effingen to Malm, Fig. 1) to values of approximately 7.4 and 7.6 towards the Malm and Keuper aquifers, respectively. Simulated redox conditions are almost stable with pe values around −2.8 at the centre and of −3.2 towards the aquifers. Corresponding EH values range from −170 to −190 mV. As can be seen in Fig. 2, simulations match well with the profiles of the major cations and anions. With relative Root Mean Square Errors (rRMSE) between 5 %–16 %, simulated pore water chemistry profile coincides well with measured data, and thus is considered as initial condition for the simulation of uranium migration.
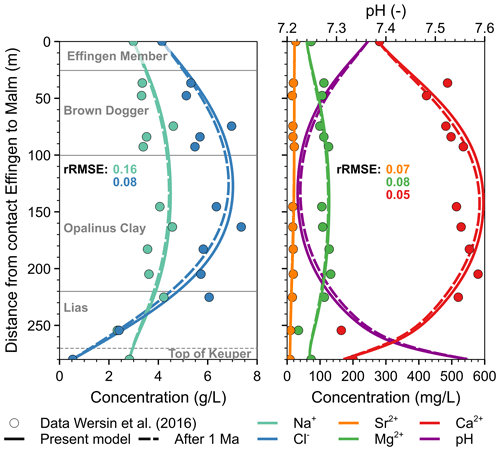
Figure 2Simulated profiles of major cations and anions (solid lines) coincide with measured concentrations (dots, Wersin et al., 2016) with a maximum deviation of 16 %. Dashed lines indicate geochemical conditions after a simulation time of 1 Ma. rRMSE = relative Root Mean Square Error.
In the geochemical system of the Opalinus Clay, iron is mainly present as Fe(II), sulphur as S(VI) and uranium as U(VI). As can be seen from Table 2, speciation of iron is dominated by Fe2+, of sulphur by and of uranium by the ternary complex Ca2UO2(CO3)3). Besides sulphate, the speciation of sulphur is characterised by complexes with the main cations, sodium, calcium and magnesium. In addition to the neutral ternary complex, uranium also forms anionic complexes with calcium, magnesium and carbonate. Fe(III), S(-II) and U(IV) species make up < 1 %, and thus are considered as zero.
3.2 Uranium migration decreases with source term concentration
Uranium diffusion and sorption processes are modelled for a simulation time of one million years using geochemical and mineralogical data from the Opalinus Clay at Schlattingen, which is close to the Swiss target area. Different source term concentrations were used in the scenarios to consider the effect of the multi-barrier system on the release of radionuclides from a highly radioactive waste disposal site. Furthermore, resulting migration lengths are compared with diffusion simulations using an experimentally determined Kd value for uranium in Opalinus Clay (Joseph et al., 2013b) and the maximum source term concentration of 10−6 mol L−1 (Hennig, 2022) as well as with a previous simulation study for the Mont Terri system (Hennig and Kühn, 2022). Simulated migration lengths of uranium correspond to the distance once the natural background concentration is reached, which is approximately 2.5 nmol L−1 (Pearson et al., 2003). Depending on the source term concentration, uranium migration ranges between 7 and 16 m (Fig. 3).
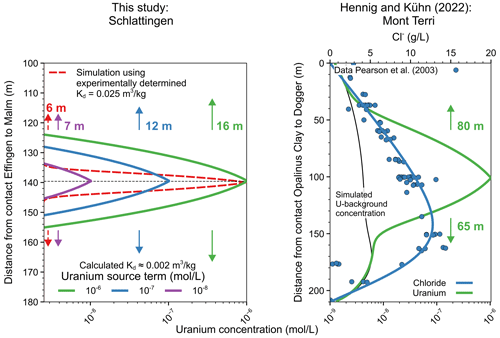
Figure 3Simulated migration lengths of uranium decrease with source term concentration. The simulation using the experimentally determined transport parameters (dashed line) underestimates uranium migration compared to the reactive transport simulations (solid lines). Only a section of the entire CRZ is shown. For comparison, the simulation results for the Mont Terri system by Hennig and Kühn (2022) are also given.
With decreasing source term concentration, uranium migrates less far through the formation (Fig. 3). Maximum migration distance is 16 m after one million years. Each decrease in the source term concentration by one order of magnitude is associated with a reduced migration of 4–5 m. However, compared to the simulations using experimentally determined transport parameters with a maximum of 6 m, uranium migration is underestimated in any case, independent of the source term concentration. Calculated and experimentally determined Kd values differ by one order of magnitude. Calculated Kd values are more or less constant across the entire CRZ. The modelled profiles of major anion and cation concentrations did not change significantly within the simulation of one million years (dashed lines, Fig. 2). Compared to the Mont Terri system with a maximum migration length of 80 m (Fig. 3), uranium migration is less by a factor of 5 in this study. In the Mont Terri system, anion and cation concentrations decrease more strongly towards the adjacent aquifers, here exemplarily shown by chloride (Figs. 3 and 2). The effect of the engineered barriers on migration lengths can be quantified with up to ±10 m.
For an application close to reality, uranium migration is quantified for different source term concentrations to consider the potential effect of the geo-engineered barriers on resulting migration lengths. Furthermore, resulting migration lengths are compared with Fick's diffusion simulations using an experimentally determined Kd value as well as with results obtained for the hydrogeochemical system at Mont Terri (Hennig, 2022). Mineralogical and geochemical data from the Opalinus Clay system at the Schlattingen borehole, which is close to the favoured disposal site in Switzerland, is used.
The hydrogeological system around the Opalinus Clay at Schlattingen is characterised by stable geochemical conditions in space and time. In contrast, at Mont Terri, concentrations of pore water components decrease towards the embedding aquifers with pronounced gradients in the profiles (Pearson et al., 2003; Mazurek et al., 2011; Hennig and Kühn, 2021b). Therefore, the profiles of cation and anion concentrations measured at Schlattingen are more or less constant within the CRZ (Wersin et al., 2016, 2018), in particular within the Opalinus Clay formation (Fig. 2). This is due to the different hydrogeological setting und paleohydrogeological evolution. At Mont Terri, hanging and footwall aquifers are flushed with meteoric water due to the Jura folding and associated erosion history (Pearson et al., 2003; Mazurek et al., 2011). Diffusive exchange between the formations is estimated to be active since 6 Ma (Mazurek et al., 2011). At Schlattingen, only the aquifer in the Keuper is active for a much shorter geological period of 0.5 Ma (Gimmi et al., 2007; Wersin et al., 2018). Furthermore, the concentration differences between groundwaters in the aquifers and pore waters in the adjacent formations are less pronounced at Schlattingen (Table 1) compared to the situation at Mont Terri (Hennig and Kühn, 2021b). Thus, pore water concentrations in the Lias decrease only slightly at Schlattingen (Fig. 2). The shape of the modelled pH and calcium profiles (Fig. 2) can be explained by the boundary conditions with respect to the different values for the fixed pCO2 (Table 1) combined with the applied mineral equilibria. Further, the results indicate that geochemical conditions do not change significantly during the simulation time of one million years (dashed lines, Fig. 2). However, it has to be noted that this is a rough estimate based on our simplified conceptual model and only valid for the applied boundary conditions. The picture will change, for instance, in case the Malm aquifer is flushed with meteoric water with lower ionic strength. Accordingly, geochemical gradients are less pronounced in this study for Schlattingen compared to Mont Terri due to the different hydrogeological setting. The system stays stable over geological time scales as long as the groundwater composition in the Malm and Keuper do not change significantly.
The maximum uranium migration distance is 16 m after one million years. Simulations conducted for the same pCO2 of 10−2.3 bar used in this study showed for the geochemical Opalinus Clay system at Mont Terri migration distances between 12–20 m depending on the litho-facies, e.g. shaly or carbonate-rich (Hennig et al., 2020). For the same source term concentration of 10−6 mol L−1, the presented results fit well into this range. In contrast, taking into account the hydrogeological system at Mont Terri and associated gradients in pore water concentrations, the maximum migration length of uranium was 80 m within one million years (Hennig and Kühn, 2022). This emphasises the potentially huge impact of the hydrogeological system. Instead, for the present study, the Kd values are constant during the simulations and along the migration path due to the stable geochemical conditions. Accordingly, observed migration lengths strongly depend on spatial and temporal variation of hydrogeological and geochemical conditions within the CRZ. Further, stable geochemical conditions, e.g. without or with low concentration gradients, enable application of the Kd approach to quantify radionuclide migration since sorption capacity does not change within such a system. Nevertheless, such Kd values are system specific for the geochemical conditions. Using experimentally determined transport parameters underestimates migration by ±10 m (Fig. 3). This is related to the difference in pCO2 between laboratory (10−3.4 bar) and in-situ (10−2.3 bar). With increasing pCO2, uranium forms more ternary complexes in solution (Table 2) what, in turn, decreases sorption (Hennig et al., 2020). Consequently, the geochemical conditions present in-situ must always be determined. Lower geochemical gradients are associated with shorter migration lengths into the host rock, and therefore are to be favoured for a safe disposal of highly radioactive waste in argillaceous formations.
A reduced source term concentration resulting from geo-engineered barriers leads to shorter migration lengths. Theoretically, the multi-barrier system, which in common concepts consists of metal canister and tunnel filling made of bentonite (Nagra, 2002; IAEA, 2003), shall minimize the release of radionuclides from the spent fuel as well as their migration into the host rock. This effect is assessed in scenarios by simply reducing the source term concentration from 10−6 to 10−7 and 10−8 mol L−1 (Fig. 3). The simulations are based on Fick's law, and thus diffusive transport depends on the diffusion coefficient, which is constant throughout the modelling domain, and the concentration gradient. Therefore, it is obvious from a numerical point of view that with decreasing source term concentration, and hence reduced gradient, less uranium is transported leading to shorter migration lengths overall. As shown in our study, this effect can be quantified with ±5 m for each decrease in the source term concentration of one order of magnitude. Since the geochemical conditions at Schlattingen are stable in space and time, the effect of the geo-engineered barriers can be estimated by the application of the Kd approach.
In a real case application, migration lengths would further diminish. All simulations are conducted one-dimensional, and thus perpendicular to the bedding, and with a constant, or in other words unlimited, uranium source term concentration. It is known that argillaceous formations like Opalinus Clay are anisotropic with respect to their tortuosity as a result of the preferential orientation of the clay platelets during deposition and compaction (Van Loon et al., 2004). As a result, diffusive transport is also anisotropic, whereby transport parallel to the bedding is faster due to a lower tortuosity. For instance, anisotropic diffusion of HTO, chloride and sodium can differ by a factor of 4–6 for the Opalinus Clay (Van Loon et al., 2004). This, in turn, would further decrease the source term concentration, and hence reduce the gradient and resulting migration lengths. Accordingly, uranium migration perpendicular and parallel to the bedding should be considered. However, as far as of the authors knowledge there is no diffusion experiment with uranium in Opalinus Clay parallel to the bedding available in the literature. Therefore, only estimates based on the knowledge from other radionuclides with smaller complexes can be made. It is questionable, whether the anisotropy factor for the De can be easily transferred to uranium. Therefore, this aspect was not further investigated in this study. Another important aspect is that the source term has a defined total amount of uranium and is not unlimited. Moreover, the source term concentration might change with time due to the geochemical changes within a repository (Ewing, 2015). However, there is no model available for the migration of uranium in the near-field that could be used as input for our simulations. Nevertheless, these points need to be kept in mind when assessing the simulation results. Consequently, diffusion perpendicular and parallel to the bedding as well as the geochemical interaction of uranium in the near-field would affect the source term, and hence resulting migration lengths.
Uranium migration for a close to real case situation is quantified using input data from the deep geothermal borehole Schlattingen, which is near the targeted area in Switzerland. For this, the present-day pore water chemistry profile at Schlattingen is modelled first and used in a second step as initial condition for the subsequent simulation of uranium migration for one million years. In order to integrate the effect of the multi-barrier system, reduced source term concentrations are used. In the Schlattingen area, the Opalinus Clay is tectonically undeformed compared to the Mont Terri anticline and represents the geochemical and temperature conditions at the favoured disposal depth. Within the 280 m thick CRZ, which is embedded between the aquifers in the Malm and Keuper, the geochemical conditions are more or less constant with a slight decrease towards the Keuper aquifer. Measured profiles of cation and anion concentrations are confirmed by the simulations. Resulting migration lengths are compared with simulations using experimentally determined transport parameters and for the geochemical system at Mont Terri.
Migration lengths resulting from reactive transport simulations strongly depend on spatial and temporal variation of the hydrogeological and geochemical conditions within the CRZ. At Mont Terri, geochemical conditions change towards adjacent aquifers. This is associated with a decrease in sorption capacity, and hence farther migration of uranium with a maximum of 80 m. In contrast, the geochemical conditions at Schlattingen are stable with a maximum migration distance of 16 m. Consequently, no or low concentration gradients are to be favoured for a safe disposal of highly radioactive waste in argillaceous formations.
Reduced source term concentration resulting from geo-engineered barriers leads to shorter migration lengths. Diffusion processes are governed, among others, by the concentration gradient along the transport pathway. Therefore, a lower gradient as established by the multi-barrier system is associated with less diffusion. Migration lengths of uranium decreased from 16 m for the maximum source term concentration (10−6 mol L−1) to 7 m for the lowest concentration (10−8 mol L−1). Thus, each decrease in the source term concentration by one order of magnitude is associated with a reduced migration of 4–5 m. For stable geochemical conditions, such as present at Schlattingen, this effect can be estimated by the application of the Kd approach.
We conclude that the hydrogeological system must always be considered in safety assessments since adjacent aquifers have a major impact on the pore water geochemistry, and hence sorption processes. This can be seen from the comparison between Mont Terri and Schlattingen for the example of uranium migration in the Opalinus Clay. In relation to the simulations using experimentally determined sorption parameters, migration is underestimated in any case, and therefore sorption processes always need to be determined for the in-situ geochemical conditions. Since sorption processes, and hence migration lengths, are mainly governed by the geochemistry, the results might also be relevant for other argillaceous formations with similar pore water geochemistry.
The applied geochemical code as referenced within the Methods part of the manuscript can be downloaded from https://www.usgs.gov/software/phreeqc-version-3 (USGS, 2021). The input scripts required to reproduce the presented simulations can be directly obtained by contacting the corresponding author.
All input data is referenced within the Methods part of the manuscript (Table 1 and Fig. 1).
Conceptualization of the manuscript was done by both authors. The software maintenance, investigation as well as original draft writing was conducted by TH. The supervision and funding acquisition was done by MK. Both authors were responsible for reviewing and editing the manuscript and all authors have read and agreed to the published version of the manuscript.
The contact author has declared that neither of the authors has any competing interests.
Publisher's note: Copernicus Publications remains neutral with regard to jurisdictional claims made in the text, published maps, institutional affiliations, or any other geographical representation in this paper. While Copernicus Publications makes every effort to include appropriate place names, the final responsibility lies with the authors.
This article is part of the special issue “European Geosciences Union General Assembly 2023, EGU Division Energy, Resources & Environment (ERE)”. It is a result of the EGU General Assembly 2023, Vienna, Austria, 23–28 April 2023.
The authors would like to thank swisstopo, the Mont Terri Project and the Helmholtz-Zentrum Dresden-Rossendorf e.V. (HZDR) for providing data and fruitful discussions.
Supported within the funding programme “Open Access Publikationskosten” Deutsche Forschungsgemeinschaft (DFG, German Research Foundation) – Project Number 491075472.
The article processing charges for this open-access publication were covered by the Helmholtz Centre Potsdam – GFZ German Research Centre for Geosciences.
This paper was edited by Johannes Miocic and reviewed by two anonymous referees.
Aubourg, C., Kars, M., Pozzi, J. P., Mazurek, M., and Grauby, O.: A magnetic geothermometer in moderately buried shales, Minerals, 11, 957, https://doi.org/10.3390/min11090957, 2021. a
Davies, C. W. and Shedlovsky, T.: Ion Association, J. Electrochem. Soc., 111, 85C, https://doi.org/10.1149/1.2426129, 1964. a
Ewing, R. C.: Long-term storage of spent nuclear fuel, Nat. Mater., 14, 252–257, https://doi.org/10.1038/nmat4226, 2015. a
Gimmi, T., Waber, H. N., Gautschi, A., and Rübel, A.: Stable water isotopes in pore water of Jurassic argillaceous rocks as tracers for solute transport over large spatial and temporal scales, Water Resour. Res., 43, W04410, https://doi.org/10.1029/2005WR004774, 2007. a, b, c, d, e, f, g
Grenthe, I., Gaona, X., Plyasunov, A. V., Rao, L., Runde, W., Grambow, B., Konings, R. J. M., Smith, A. L., and Moore, E. E.: Second update on the chemical thermodynamics of uranium, neptunium, plutonium, americium and technecium, Chemical Thermodynamics no. 14, OECD Nuclear Energy Agency, Boulogne-Billancourt, France, https://www.oecd-nea.org (last access: 19 September 2023), 2020. a
Hennig, T.: Uranium migration in the Opalinus Clay quantified on the host rock scale with reactive transport simulations, Dissertation, University of Potsdam, https://publishup.uni-potsdam.de/frontdoor/index/index/docId/55270 (last access: 24 May 2023), 2022. a, b, c, d
Hennig, T. and Kühn, M.: Surrogate model for multi-component diffusion of uranium through Opalinus Clay on the host rock scale, Appl. Sci., 11, 786, https://doi.org/10.3390/app11020786, 2021a. a
Hennig, T. and Kühn, M.: Potential uranium migration within the geochemical gradient of the Opalinus Clay system at the Mont Terri, Minerals, 11, 1087, https://doi.org/10.3390/min11101087, 2021b. a, b, c, d, e, f, g
Hennig, T. and Kühn, M.: Reactive transport simulations of uranium migration in the Opalinus Clay depend on ion speciation governed by underlying thermodynamic data, Adv. Geosci., 58, 11–18, https://doi.org/10.5194/adgeo-58-11-2022, 2022. a, b, c, d, e, f, g, h, i
Hennig, T., Stockmann, M., and Kühn, M.: Simulation of diffusive uranium transport and sorption processes in the Opalinus Clay, Appl. Geochem., 123, 104777, https://doi.org/10.1016/j.apgeochem.2020.104777, 2020. a, b, c, d, e, f, g, h, i
IAEA: Scientific and technical basis for the geological disposal of radioactive wastes, Technical Report Series no. 413, International Atomic Energy Agency, Vienna, Austria, https://www.iaea.org/publications/search/type/technical-reports-series (last access: 12 October 2023), 2003. a, b, c
IAEA: The safety case and safety assessment for the disposal of radioactive waste, Safety Standards Series – Specific Safety Guide no. SSG-23, International Atomic Energy Agency, Vienna, Austria, https://www.iaea.org/publications/8790/the-safety-case-and-safety-assessment-for-the-disposal-of-radioactive-waste (last access: 12 October 2023), 2012. a
Joseph, C., Schmeide, K., Sachs, S., Brendler, V., Geipel, G., and Bernhard, G.: Sorption of uranium(VI) onto Opalinus Clay in the absence and presence of humic acid in Opalinus Clay pore water, Chem. Geol., 284, 240–250, https://doi.org/10.1016/j.chemgeo.2011.03.001, 2011. a, b
Joseph, C., Stockmann, M., Schmeide, K., Sachs, S., Brendler, V., and Bernhard, G.: Sorption of U(VI) onto Opalinus Clay: effects of pH and humic acid, Appl. Geochem., 36, 104–117, https://doi.org/10.1016/j.apgeochem.2013.06.016, 2013a. a
Joseph, C., Van Loon, L. R., Jakob, A., Steudtner, R., Schmeide, K., Sachs, S., and Bernhard, G.: Diffusion of U(VI) in Opalinus Clay: influence of temperature and humic acid, Geochim. Cosmochim. Ac., 109, 74–89, https://doi.org/10.1016/j.gca.2013.01.027, 2013b. a, b, c, d, e, f
Lerouge, C., Grangeon, S., Claret, F., Gaucher, E., Blanc, P., Guerrot, C., Flehoc, C., Wille, G., and Mazurek, M.: Mineralogical and isotopic record of diagenesis from the Opalinus Clay formation at Benken, Switzerland: implications for the modeling of porewater chemistry in a clay formation, Clay. Clay Miner., 62, 286–312, https://doi.org/10.1346/CCMN.2014.0620404, 2014. a, b
Lerouge, C., Maubec, N., Wille, G., and Flehoc, C.: Geochemical Data (GD) experiment: analysis of carbonate fraction in Opalinus Clay, Technical Note TN 2014-92, Mont Terri Project, St. Ursanne, Switzerland, 2015. a
Marques Fernandes, M., Vér, N., and Baeyens, B.: Predicting the uptake of Cs, Co, Ni, Eu, Th and U on argillaceous rocks using sorption models for illite, Appl. Geochem., 59, 189–199, https://doi.org/10.1016/j.apgeochem.2015.05.006, 2015. a
Mazurek, M., Alt-Epping, P., Bath, A., Gimmi, T., Waber, H. N., Buschaert, S., De Cannière, P., De Craen, M., Gautschi, A., Savoye, S., Vinsot, A., Wemaere, I., and Wouters, L.: Natural tracer profiles across argillaceous formations, Appl. Geochem., 26, 1035–1064, https://doi.org/10.1016/j.apgeochem.2011.03.124, 2011. a, b, c
Mazurek, M., Oyama, T., Wersin, P., and Alt-Epping, P.: Pore-water squeezing from indurated shales, Chem. Geol., 400, 106–121, https://doi.org/10.1016/j.chemgeo.2015.02.008, 2015. a
Montavon, G., Ribet, S., Hassan Loni, Y., Maia, F., Bailly, C., David, K., Lerouge, C., Madé, B., Robinet, J. C., and Grambow, B.: Uranium retention in a Callovo-Oxfordian clay rock formation: from laboratory-based models to in natura conditions, Chemosphere, 299, 134307, https://doi.org/10.1016/j.chemosphere.2022.134307, 2022. a
Nagra: Project Opalinus Clay – Safety Report. Demonstration of disposal feasibility for spent fuel, vitrified high-level waste and long-lived intermediate-level waste (Entsorgungsnachweis), Nagra Technical Report NTB 02-05, Nagra, Wettingen, Switzerland, https://www.nagra.ch/en/download-centre (last access: 12 October 2023), 2002. a, b
Nagra: Entsorgungsprogramm 2021, Nagra Technical Report NTB 21-01, Nagra, Wettingen, Switzerland, http://www.nagra.ch/downloads (last access: 18 September 2023), 2021. a
Nagra: TBO Stadel-3-1: Data Report (Dossier VIII – Rock properties, porewater characterisation and natural tracer profiles), Nagra Arbeitsbericht NAB 22-01, Nagra, Wettingen, Switzerland, https://nagra.ch/downloads/ (last access: 24 May 2023), 2022. a, b
Noseck, U., Brendler, V., Britz, S., Stockmann, M., Fricke, J., Richter, C., Lampe, M., Gehrke, A., and Flügge, J.: Smart Kd-concept for long-term safety assessments: extension towards more complex applications, Tech. Rep. GRS-500, GRS and HZDR, Cologne and Dresden, Germany, ISBN 978-3-946607-85-4, 2018. a
Parkhurst, D. L. and Appelo, C. A. J.: Description of input and examples for PHREEQC Version 3 – A computer program for speciation, batch-reaction, one-dimensional transport, and inverse geochemical calculations, in: Book 6 – Modelling techniques, Chap. A43, p. 497, https://pubs.usgs.gov/tm/06/a43/pdf/tm6-A43.pdf (last access: 12 October 2023), 2013. a
Pearson, F. J., Arcos, D., Bath, A., Boisson, J.-Y., Fernández, A. M., Gäbler, H. E., Gaucher, E. C., Gautschi, A., Griffault, L., Hernán, P., and Waber, H. N.: Mont Terri Project – Geochemistry of water in the Opalinus Clay formation at the Mont Terri rock laboratory, Reports of the FOWG, Geology Series no. 5, Federal Office for Water and Geology (FOWG), Bern, Switzerland, ISBN 3-906723-59-3, 2003. a, b, c, d
Pearson, F. J., Tournassat, C., and Gaucher, E. C.: Biogeochemical processes in a clay formation in-situ experiment: part E – Equilibrium controls on chemistry of pore water from the Opalinus Clay, Mont Terri underground research laboratory, Switzerland, Appl. Geochem., 26, 990–1008, https://doi.org/10.1016/j.apgeochem.2011.03.008, 2011. a
Pekala, M., Wersin, P., Rufer, D., and Curti, E.: Geochemical Data (GD) experiment: mineralogy of carbonate and sulphate minerals in the Opalinus Clay and adjacent formations, Technical Report TR 2018-03, Mont Terri Project, 2019. a
Stockmann, M., Schikora, J., Becker, D. A., Flügge, J., Noseck, U., and Brendler, V.: Smart Kd-values, their uncertainties and sensitivities – Applying a new approach for realistic distribution coefficients in geochemical modelling of complex systems, Chemosphere, 187, 277–285, https://doi.org/10.1016/j.chemosphere.2017.08.115, 2017. a, b
Thoenen, T., Hummel, W., Berner, U., and Curti, E.: The PSI/Nagra chemical thermodynamic database 12/07, PSI Report no. 14-04, Paul Scherrer Institute, Villigen, Switzerland, https://www.psi.ch/en/les/database (last access: 12 October 2023), 2014. a
USGS: PHREEQC Version 3, Software release 3.5.0, https://www.usgs.gov/software/phreeqc-version-3 (last access: 4 September 2019), 2021. a
Van Loon, L. R., Soler, J. M., Müller, W., and Bradbury, M. H.: Anisotropic diffusion in layered argillaceous rocks: a case study with Opalinus Clay, Environ. Sci. Technol., 38, 5721–5728, https://doi.org/10.1021/es049937g, 2004. a, b
Van Loon, L. R., Müller, W., and Iijima, K.: Activation energies of the self-diffusion of HTO, 22Na+ and 36Cl− in a highly compacted argillaceous rock (Opalinus Clay), Appl. Geochem., 20, 961–972, https://doi.org/10.1016/j.apgeochem.2004.10.007, 2005. a
Wersin, P., Mazurek, M., Waber, H. N., Mäder, U. K., Gimmi, T., Rufer, D., and de Haller, A.: Rock and porewater characterisation on drillcores from the Schlattingen borehole, Nagra Arbeitsbericht NAB 12-54, Nagra, Wettingen, Switzerland, https://nagra.ch/downloads/ (last access: 24 May 2023), 2013. a
Wersin, P., Mazurek, M., Mäder, U. K., Gimmi, T., Rufer, D., Lerouge, C., and Traber, D.: Constraining pore water chemistry in a 250 m thick argillaceous rock sequence, Chem. Geol., 434, 43–61, https://doi.org/10.1016/j.chemgeo.2016.04.006, 2016. a, b, c, d, e, f, g, h, i, j, k, l, m, n, o, p, q
Wersin, P., Gimmi, T., Mazurek, M., Alt-Epping, P., Pȩkala, M., and Traber, D.: Multi-component diffusion in a 280 m thick argillaceous rock sequence, Appl. Geochem., 95, 110–123, https://doi.org/10.1016/j.apgeochem.2018.05.013, 2018. a, b, c, d, e, f, g, h, i, j, k, l, m
Wersin, P., Mazurek, M., and Gimmi, T.: Pore water chemistry of Opalinus Clay revisited: findings from 25 years of data collection at the Mont Terri Rock Laboratory, Appl. Geochem., 138, 105234, https://doi.org/10.1016/j.apgeochem.2022.105234, 2022. a