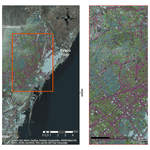
ASSET project: assessing sustainable urban drainage system (SUDS) efficiency to reduce urban runoff water contamination
Marc Teixidó
Rotman Criollo
Francesc Labad
Enric Vázquez-Suñé
María Izquierdo
María José Chesa Marro
Daniel de Castro
Increase of population in the current climate change scenario requires to ensure the quality of groundwater available as well as to control possible incomes of pollution into the subsurface system. The objective of this work was to evaluate the current quality of groundwater and the risk of infiltration of surface contaminants into the aquifers customizing the DRASTIC method including potential flood areas and areas with high risk of surface pollution.
Hydrogeological, hydrochemical and isotopic data compiled and measured show the aquifer behavior and the main attenuation and degradation of contaminants. The vulnerability to contamination of Barcelona aquifers by direct surface contaminants is relatively low considering flood areas and traffic. However, there is a relevant area where this risk is relatively high. Nevertheless, it is required further analyses considering the drainage network of the city including as well the geometry of Sustainable Urban Drainage Systems (SUDS) already installed.
The knowledge of the aquifer hydrogeological characteristics and its quality together with the identification of vulnerable to contamination areas may lead to improve the installation of SUDS, reducing the input of contaminants through these infrastructures. The implementation of this methodology aims to facilitate water users and urban managers to control their potential negative effects on the receiving water body. In addition, the outcomes of its application may be used to optimize the groundwater management in the city.
- Article
(3508 KB) - Full-text XML
- BibTeX
- EndNote
Due to the current global change, there is a need looking for improved urban water management. Especially in urban areas, where most of the population is concentrated. These high dense areas require improvements on water quantity and quality, and Barcelona city is not an exception. Barcelona City Council has been studying different alternatives to manage city water scarcity since 1994. Following these new strategies, city groundwater is currently applied for different purposes. Even more, aquifers are strategic water bodies that can be used during scarcity events for non-potable and potable water supply.
In addition, Barcelona City Council installed different green infrastructures called Sustainable Urban Drainage Systems (SUDS). These installations reduce the extreme runoff events by promoting and facilitating the recharge of the aquifers. The installation of these systems is increasing, but there is a lack of the knowledge and understanding of the quality of the water infiltrated in the aquifer and their effects on the state of the groundwater bodies of the city, which can contribute to reduce the current quality of groundwater.
It is required to evaluate the impact of the SUDS implemented in the city of Barcelona and provide improvements so that these systems could be more efficient and fulfil their purpose towards an efficient and sustainable use of water, improving the adaptation capacity of the city to the current Climate Change. The objective of this work project is to evaluate the current quality of groundwater and the risk of infiltration of surface contaminants into the aquifers by SUDS systems.
2.1 Site description
The study area is located in Barcelona city at the northeast of Spain. Four zones can be distinguished according to its hydrogeological characteristics: (1) the Collserola Mountains (mainly metamorphic materials), (2) the Llobregat delta (alluvial fans), (3) Besòs delta (alluvial fans) and (4) the Barcelona plain (alluvial fans) (Tubau et al., 2017). The hydraulic flow is dependent on the water balance, the anthropic impacts such as underground infrastructures (tunnels or car parks), and groundwater extractions (dewatering) that are distributed throughout the city. The hydraulic gradient, in general, from the mountains to the sea, crosses different geological units until arrive to the sea. In addition to the main natural recharge sources (mountains and the Besòs River), there are some anthropic sources such as losses in sewage and water supply networks, mainly that artificially recharge the aquifers, directly impacting the groundwater quality. The Collserola Mountains and the topographic highs of the alluvial plains of the Barcelona plain do not present any areas where rainwater is captured by wells and springs.
2.2 Sampling and analytical methods
Groundwater samples were collected from a total of 30 wells and piezometers, distributed throughout the study area. Wells were purged and samples collected after removing three well volumes or once flied parameters had stabilized. The physico-chemical parameters such as temperature (∘C), pH, Specific Conductance (SC, µS cm−1), Eh and dissolved oxygen (DO, mg L−1) were measured in situ inside a closed flow cell. Total alkalinity was determined in the field by acid-base titration using an Aquamerck Alkalinity kit.
Groundwater samples for general chemistry were collected in high-density polyethylene, 25 mL bottles for anions and 50 mL for cation-trace samples, previously filtered through a 0.22 µm nylon filter. Cation-tracer samples were acidified with 1 mL of 20 % diluted nitric acid for sample preservation. Anions were analysed by ion chromatography (IC) and cations by Inductively Coupled Plasma Atomic Emission Spectrometry (ICP-AES). The total trace elements concentrations were measured by inductively coupled plasma and mass spectrometry (ICP-MS). In order to differentiate arsenite (As (III)) from arsenate (As(V)) field speciation cartridges were used (Meng and Wang, 1998). Dissolved Organic Carbon (DOC) samples were collected in 30 mL glass bottles previously muffled. These samples were filtered through a 0.45 µm nylon filter and acidified with 1 mL of HCl (2N). DOC was analysed by the catalytic oxidation method at 680 ∘C using a Shimadzu TOC-V CSH instrument, with a detection limit of 0.05 mg L−1. NH and I− concentrations were analyzed with ion selective electrodes Orion 9512, with an error of ±2 %.
2.3 Data compilation
Hydrogeological information collected from multiple sources, such as scientific investigations and civil works conducted in the city, were homogenized and integrated into HYDORGIS (Velasco et al., 2013). This GIS-based platform facilitate and improve the reporting of results of groundwater quality and quantity. For further analyses, this platform is connected with external software to implement further water mix analysis (MIX, Carrera et al., 2021), hydrochemical balance (Easy_Quim, Serrano-Juan et al., 2020), and for the interpretation of hydraulic parameters (MJ-Pumpit, Criollo et al., 2016) (see Fig. 1).
2.4 Vulnerability assessment
Hydrogeological data included into the HYDORGIS platform was used for the vulnerability assessment. The DRASTIC method (Aller et al., 1987) was adapted in order to evaluate which places of the city may be an additional source of infiltration of surface pollutants. The evaluation was performed including potential areas to have flood areas, puddles, and areas with high risk of surface pollution coming from traffic. Puddles were included using the Cloudburst method, obtaining bluespots based on a hydrologically adapted digital terrain model (Balstrøm, 2020). This method has been widely applied in cities of Denmark, Sweden or and Germany. A 5 m resolution DEM is used to locate these terrain depressions as well as Barcelona's urban barriers. To find this terrain depressions together with Barcelona urban barriers, a DEM with 5 m resolution.
Surface pollution was included indirectly following the width and slope of the streets. Streets with high width and less slope would increase the risk to lay pollutants and, hence, have impact on the water cycle. This variable was divided into three ranges: less than 10 m; between 10 and grater than 20 m.
3.1 General hydrochemistry
According to the location and hydrogeological units of the samples, as well as the spatial distribution of the major ions, samples were grouped into 3 groups; Besòs River (BR), Barcelona Plain (BP) (topographically lower part of the Barcelona Plain, Llobregat delta and Montjuïc), and High Zone (HZ) (Fig. 2).
The electrical conductivity (EC) of these waters ranged from 500 to 5500 µS cm−1, with a mean of 1448±490 µS cm−1. Some samples had conductivities lower than 1000 µS cm−1, but most have values between 1000–2000 µS cm−1, with an anomalous sample (HJOM) that has a conductivity of 5510 µS cm−1. Higher EC values are observed by the coast and in the Barcelona plain at the districts of Sants-Montjuic, Eixample and Ciutat Vella, which is consistent with the fact that these samples are located in highly populated neighborhoods and have had a longer residence time. However, there are also some samples with lower conductivities (<1200 µS cm−1) in these sectors, indicating a high degree of heterogeneity in the aquifer. Towards the higher zone (Horta – Guinardó and Nou Barris) conductivities are relatively lower, consistent with the fact that these samples are located in less populated neighborhoods and have had a shorter residence time.
The pH range is quite narrow, slightly more alkaline pH values (6.5–7.3) were observed towards the Besòs River, while the Barcelona plain and the Llobregat River slightly more acidic (5.5–6.5). In general, these waters are in the pH range of groundwater, the slight differences can be attributed to the interaction with host materials (e.g., carbonates tend to increase the pH) as well as to anthropic contributions.
Temperature of these waters mostly ranges between 20–22 ∘C. The lowest temperatures (18 ∘C) are located in 2 sites in the higher zone, the highest temperatures (22–25 ∘C) are observed at the boundaries of the aquifer formations, e.g., at the Besòs River with the Barcelona Plain. These variations may be due to the proximity to the recharge source as well as to anthropogenic inputs, in particular industrial and/or domestic discharges (e.g. buildings pumping groundwater for cooling/heating systems).
The descriptive statistics and box-and-whisker diagrams of the major ions are shown in Fig. 3. Regarding the anions, the most abundant is HCO, with an average of 333 mg L−1, followed by Cl− (218 mg L−1), SO (196 mg L−1) and lastly NO (53 mg L−1). However, the maximum of chloride (1092 mg L−1) exceeds the maximum of bicarbonate (616 mg L−1). Regarding the cations, the most abundant is Ca2+, with an average of 155 mg L−1, followed by Na+ (119 mg L−1), Mg2+ (46 mg L−1) and finally K+ (7 mg L−1). The maximum of Na+ (453 mg L−1) exceeds the maximum of Ca2+ (393 mg L−1). The higher average concentrations of HCO and Ca2+ are consistent with water-rock interaction processes (dissolution of carbonate materials with the presence of calcite). The maximum concentrations for most of these ions are repeated for the same sample (HJOM) which also presented the highest E.C., that could be due to a point-source contamination. The WEPO and DFI2 samples also presented unexpectedly high concentrations for some ions, which are probably also due to point-source contamination (industrial discharge for DFI2 given its location in an industrial sector or saline intrusion for WEPO given its proximity to the coast and in particular the pumping carried out in Glòries square DFIR2).
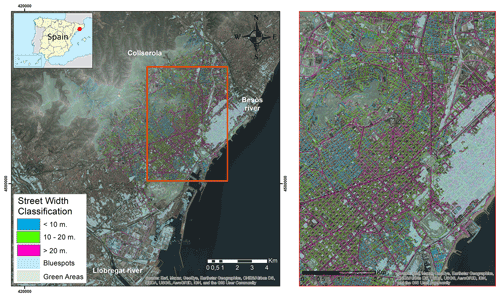
Figure 6Additional information included in the DRASTIC method to evaluate the risk of infiltration of surface pollutants into the aquifers of the city. Streets width classification as 3 ranges: <10 (greenblue); 10–20 (yellowgreen); >20 m (redmagenta) was used as indirect indicator of traffic. Bluespots (light blue polygons) shows potential areas of puddles during high precipitation events (Balstrøm, 2020). Source for basemap: © ArcGIS (ESRI).
The concentrations of Cl− are mostly between 100–250 mg L−1, with the highest concentrations by the coast (250–500 mg L−1) which could indicate some marine contribution. Lower concentrations (<100 mg L−1) are distributed mostly towards the higher zone and the TRME sample (Barcelona Plain). The HJOM sample as already observed with the EC, presents an anomalous value of 1092 mg L−1 of Cl−, probably due to a one-time contamination episode. More than 50 % of the HCO samples presented concentrations between 250–350 mg L−1 without a particular spatial distribution pattern. Those with lower concentrations (<250 mg L−1) are located towards the higher zone, however in this area there is also a sample (FVIL) with a higher concentration (450–616 mg L−1), as well as the sample HJOM and DFI2. In general, SO concentration of samples present values between 200–300 mg L−1. The lowest concentrations of this ion (<200 mg L−1) occur towards Besòs River and higher zone. The highest concentrations of SO occur in the samples DFI2 and VITP with 300 and486 mg L−1 respectivetly. High values of this ion in the urban area could be associated with old dumps of construction materials, the dissolution of evaporitic materials and/or domestic detergents associated with wastewater. Sulfate oxidation processes could occur in Collserola and in its sedimentary deposits. Regarding NO, high concentrations were observed in most of the samples (50–200 mg L−1). Most high values occurred in the Barcelona Plain and the High Zone. Nitrate contributions in an urban sector may be associated with seepage from pipes and/or septic tanks depending on the location of the samples. The fact that towards the Besòs River, there are lower values of SOand NO could be associated with redox processes such as sulfate reduction and/or denitrification, as well as with few samples that also presented lower values of SO and NO . Ca2+ presents concentrations between 100–200 mg L−1 for most of the samples, near the coast higher values were measured (200–300 mg L−1) and again the HJOM sample presents the highest concentration (393 mg L−1). Mg2+ presents a quite clear distribution pattern with the highest concentrations (40–80 mg L−1) towards the Barcelona Plain except for the 2 samples located in Montjuïc (20–40 mg L−1) and the anomalous sample HJOM (155 mg L−1). Towards the Besòs River and High Zone the concentrations are lower (<40 mg L−1). Na+ has lower concentrations (<100 mg L−1) towards the High Zone, while towards the Besòs River and Barcelona Plain concentrations are between 100–200 mg L−1; the anomalous samples HJOM and WEPO (towards the coast) presented the highest concentrations (200–453 mg L−1). Regarding K+ which presented low concentrations throughout the territory, the highest values (10–15 mg L−1) is in the area of the Besòs River, while towards the High Zone and Barcelona Plain those concentration are generally <5 mg L−1, the samples with the highest values (15–28 mg L−1) correspond to WEPO and DFI2, which are located towards the coast.
It should be noted, that the TRME sample located towards Barcelona Plain presented lowest concentrations of the major ions except for K+, which could be associated with a low residence time or some other factor. Its composition is more similar to the samples of the High Zone. From the box and whisker plots (Fig. 3) and the spatial distribution of the major ions, it was observed that there are samples (HJOM, WEPO, FVIL, DFI2, VITP) that present anomalously high concentrations for some ions, which would indicate an extra contribution (marine intrusion, sewage filtration, urban/industrial discharges, among others).
Figure 4 shows the Piper diagram of the samples, which represents the relative composition of these waters. Most of the samples present a mixed composition; however, calcium can be identified as the dominant cation, which is probably due to water-rock interaction processes, considering the abundance of carbonate deposits (e.g., calcite). The dominant anion corresponds to bicarbonate (Besòs River and High Zone) and chloride (Barcelona Plain). The samples showing chloride composition in general also presented a higher mineralization, which can be attributed to a longer travel times in the aquifer.
In addition, isotope analyses of the sulfate molecule were performed. The δ18O values are in a range of 8 ‰–12 ‰, while the δ34S between 5 ‰–14 ‰: With these values it could be ruled out the contribution by residues of construction materials that according to Vázquez-Suñé (2003) varies between −14 to 0.4 for the δ18O. Vázquez-Suñé et al. (2016) and Jurado Elices (2013) obtained isotopic values of the sulfate molecule for the possible recharge end-members of the system, where the wastewater presented heavier isotopic values than the distribution waters. This is associated with the contribution of domestic detergents and sulfate reduction processes associated with waters with high organic matter content. Figure 5 shows the graph of the sulfate isotopes for the groundwater samples, together with the isotopic value for the sewage and the trend line of the seawater. It is observed that the samples plot quite close to the sewage field, consistent with observations made on the recharge waters (Vázquez-Suñé et al., 2016). In addition, some samples are aligned with the seawater line, indicating contributions from marine sediments (e.g., evaporites). On the other hand, it is observed that the alignment of the samples would indicate sulfate reduction processes, in particular, samples DTA1 and FTAU in the lower part of the river presented the heaviest values of 34δS, which could be associated with an important contribution of wastewater from both the river and industries.
3.2 Vulnerability
The resolution of the vulnerability map is constrained by the pixel resolution of the DEM (Digital Elevation Model) applied of 5 m. Nevertheless, this resolution is enough to elucidate vulnerability zones of the city concerning infiltration of pollutants into the aquifers. River-aquifer connection is not studied and integrated in the scope of this work.
Cloudburst method shows flood areas, or puddles, increasing while the slope of the city is reducing until arrive to the coastline, mainly in the Llobregat (where the airport and the harbor are located) and at the boundaries of the Besòs River with the Plana de Barcelona groundwater bodies. This area is the densest zone of roads with more than 10 m width and Bluespots (Fig. 6). According with the hydrogeological and hydrochemical analysis, this area presents a relevant infiltration by industry and domestic leackages.
The objective of this work was to evaluate the current quality of groundwater and the risk of infiltration of surface contaminants into the aquifer customizing the DRASTIC method including potential flood areas and areas with high risk of surface pollution.
Hydrogeological, hydrochemical, and isotopic data compiled and measured indicate different groundwater types following groundwater flux. RedOX environmental conditions are the major attenuation factor of most of the contaminants into the aquifers. The aquifer heterogeneity leads to different travel times and it may impact on the attenuation and degradation of most of the contaminants found in groundwater samples.
In general, considering flood areas and traffic, as well as the existing sewer system and other underground stormwater storage systems, the vulnerability of aquifer contamination by direct surface contaminants in the city is relatively low. The boundary of the Besòs River and the Barcelona plain aquifers presents the highest risk to infiltrate pollutants because of characteristics of the city (i.e., slope, street width, geology, deep of groundwater levels) and land uses in the area. Nevertheless, it is required further analyses considering the sanitary and drainage network of the city including as well the geometry of SUDS already installed. It may increase the understanding of which role SUDS play in the hydraulic balance of the city aquifers and how they attenuate contaminants before arrival into the aquifers.
The knowledge of the aquifer characteristics and its quality together with the control of vulnerable areas can lead to improve the installation of SUDS reducing the input of contaminants trough these infrastructures. The implementation of this methodology facilitates water utility engineers and urban planners, which control the possible effects of this merging. The outcomes of its application may be used to optimize the groundwater management in the city.
The code can be provided by the corresponding authors upon request.
All raw data can be provided by the corresponding authors upon request.
MT, LS and RC are planned and ejecuted the field campaign; MT and FL analysed the data; EVS and MI supervised all the work related with ASSET project; MJCM and DdC facilitated access to sampling points; LS, MT, RC, FL, EVS, MI, MJCM and DdC wrote the manuscript draft and reviewed and edited the manuscript.
The contact author has declared that none of the authors has any competing interests.
Publisher’s note: Copernicus Publications remains neutral with regard to jurisdictional claims in published maps and institutional affiliations.
This article is part of the special issue “Quality and quantity issues in urban hydrogeology (EGU2022 HS8.2.8 session)”. It is a result of the EGU General Assembly 2022, Vienna, Austria, 23–27 May 2022.
The authors thank the support from the Barcelona city council through the Award for Scientific Research into Urban Challenges in the City of Barcelona 2020 (reference codes: 21S04651, 21S04652 and 21S04653). The authors would like to thank the European Commission, the Spanish Foundation for Science and Technology (FECYT) and Spanish State Research Agency (AEI) for funding in the frame of the collaborative international consortium (URBANWAT) financed under the 2018 Joint call of the WaterWorks2017 ERA-NET Cofund. This ERA-NET is an integral part of the activities developed by the Water JPI. Additionally authors would also thank the Ministry of Science, Innovation and Universities, for funding the project UNBIASED (Ref: RTI2018-097346-B-I00) under the 2018 call of the “Proyectos de I+D Retos Investigación”.
This research has been supported by Barcelona city council through the Award for Scientific Research into Urban Challenges in the City of Barcelona 2020 (reference codes: 21S04651, 21S04652 and 21S04653).
We acknowledge support of the publication fee by the CSIC Open Access Publication Support Initiative through its Unit of Information Resources for Research (URICI).
This paper was edited by Miao Jing and reviewed by Laura Balzani and one anonymous referee.
Aller, L., Bennett, T., Lehr, J., Petty, R., and Hackett, G.: DRASTIC: a standardized system for evaluating groundwater pollution potential using hydrogeologic settings: U.S. environmental protection agency report 600/2-87/035, p. 622, 1987.
Balstrøm, T.: Coping with Cloudbursts 2020. An intro to bluespot modeling, https://storymaps.arcgis.com/stories/bc7fdca84e6c48c08e24b951da374c42 (last access: August 2022), 2020.
Carrera, J., Vázquez-Suñé, E., Castillo, O., and Sánchez-Vila, X.: A methodology to compute mixing ratios with uncertain end-members, Water Resour. Res., 40, 1944–7973, https://doi.org/10.1029/2003WR002263, 2021.
Criollo, R., Velasco, V., Vázquez-Suné, E., Serrano-Juan, A., Alcaraz, M., and García-Gil, A.: An integrated GIS-based tool for aquifer test analysis, Environ. Earth Sci., 75, 1–11, https://doi.org/10.1007/s12665-016-5292-3, 2016.
Serrano-Juan, A., Criollo, R., Vázquez-Suñè, E., Alcaraz, M., Ayora, C., Velasco, V., and Scheiber, L.: Customization, extension and reuse of outdated hydrogeological software, Geol. Acta, 18, 1–13, https://doi.org/10.1344/GeologicaActa2020.18.9, 2020.
Jurado Elices, A.: Occurrence and fate of emerging contaminants in urban groundwater. A case study: Barcelona. Tesi doctoral, UPC, Departament d'Enginyeria del Terreny, Cartogràfica i Geofísica, http://hdl.handle.net/2117/95622, 2013.
Meng, X. and Wang, W.: Speciation of arsenic by disposable cartridges, in: Book of posters of the third international conference on arsenic exposure and health effects, Society of Environmental Geochemistry and Health, University of Colorado at Denver, Denver, CO, 1998.
Tubau, I., Vázquez-Suñé, E., Carrera, J., Valhondo, C., and Criollo, R.: Quantification of groundwater recharge in urban environments, Sci. Total Environ., 391–402, https://doi.org/10.1016/j.scitotenv.2017.03.118, 2017.
Vázquez Suñé, E.: Urban Groundwater. Barcelona City Case Study. Tesi doctoral, UPC, Departament d'Enginyeria del Terreny, Cartogràfica i Geofísica, ISBN 8468892858, http://hdl.handle.net/2117/93544, 2003.
Vázquez-Suñé, E., Criollo, R., Tubau, I., Jurado, A., Ansón, I., Soler, A., Carrera, J., Chesa, M. J., Burdons, S., Arandes, R., and Iglesias, M.: Hidroquímica i contaminació dels aqüífers urbans de l'àrea de Barcelona. Geoquímica ambiental a Catalunya, Barcelona, Chapter 12, ISBN 9788439394716, http://hdl.handle.net/10261/140967, 2016.
Velasco, V., Vázquez-Suñé, E., Criollo, R., Alcaraz, M., Serrano, A., García-Gil, A., Tubau, I., Gogu, R., and Gaitanaru, D.: GIS-based Hydrogeological Database and Analysis Tools. INFOCOMP 2013: The Third International Conference on Advanced Communications and Computation. IARIA, ISBN 978-1-61208-310-0, 2013.