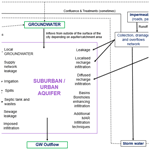
Protection of peri-urban groundwater catchments: a multi-tracer approach for the identification of urban pollution sources
Laura Balzani
Philippe Orban
Serge Brouyère
Groundwater catchment located in peri-urban areas may be impacted by many pollutants coming from different types of point or diffuse sources such as accidental spills, continuous hidden leaks in drainage networks, old landfills, treated/untreated wastewater and watercourses. In the scope of the CASPER project, a new methodological approach has been developed based on field survey and interpretation of the collected data in order to distinguish between the different sources of contamination and mixtures of pollutants. First, the groundwater catchment area corresponding to the land surface perimeter in which abstracted groundwater is recharged is determined and characterised in hydrogeological terms. The possible sources of pollution are identified. In a second step, a groundwater and surface water monitoring survey is established, and water samples are collected focusing on a combination of physicochemical parameters and set of various hydrochemical indicators. In particular, different stable isotopes are considered. The NO and Boron stable isotopes are used to distinguish between inputs linked to urban effluents, agricultural fertilisers and manure. Stable isotopes of SO are used to distinguish between sulphide minerals oxidation, sulphur-carbon compounds mineralisation, lixiviation and human pollution. Moreover, the occurrence of specific molecules like pharmaceutical and lifestyle products (carbamazepine, caffeine, etc.) are used as effective tracers of anthropogenic contamination. Microbiological analyses are also undertaken to identify microbial populations associated with specific sources of pollution or specific biochemical reactions occurring in soil and groundwater. The resulting hydrochemical dataset is then processed using multivariate and clustering analyses. In this context, the objective here is to describe the rigorous methodological approach to assess pollution sources and to illustrate the first steps of this process using a case study corresponding to a groundwater catchment is a chalk aquifer in Western Belgium.
In Wallonia (south part of Belgium), approximately 80 % of drinking water supply is provided by groundwater catchments. Many of these are located in rural areas and are therefore most often threatened or impacted by pollutants of agricultural origin, such as nitrates and pesticides. However, another significant portion of groundwater catchments are in urbanised environments (residential areas, economic activity zones, proximity to waterways, etc.). These peri-urban areas can be associated with a wide variety of pollution sources ranging from accidental punctual spills to more continuous pollution, hidden and sometimes larger-scale pollution linked to faults/leakage in drainage networks, known or unknown landfills, treated or untreated housing and industrial wastewater, storage systems for products for private or industrial use (fuel oil tanks, etc.). These catchments may also interact strongly with superficial watercourses. The problem linked to the complexity of those urban/sub-urban catchments points out the diversity of land use occupation reflected in the relevant diversity of pollution sources linked to that.
In this context, the CASPER project aims to develop an integrated operational methodology to optimise the protection of groundwater resources and water catchments from urban pollution in their recharge areas. More specifically, this requires (1) identifying their origin(s) which is (are) not always known (2) characterising their composition and their importance in terms of pollutant load, (3) assessing the current and future risks for the environment, (4) assessing the current and future risks they represent for the catchments and, as a result, the need for remediation. To do so, firstly the challenge is on being able to discriminate the different pollution sources using a combination of specific tracers and approaches (such as isotopes ratio (Nikolenko et al., 2018; Widory et al., 2005), occurrence of pharmaceautical substances (Neufcourt, 2017), Gd analysis (Boester and Rüde, 2020; Petelet-Giraud et al., 2009) etc.). And then, on being able to build a decision-making reference system to prioritize pollutions and concerned remediation measures.
This project takes place in the well-known context of the modification of the “urban water cycle”, mainly influenced by the increase in the number of impermeable surfaces due to the construction of houses, traffic lanes, car parks, etc.… and the increase in – antropic sources (drinkwater supply networks – domestic water consumption, despite the use of water-efficient machines, due to the number of households/house and comforts like swimming pools and gardens) and sewage. Nowadays there are many different inputs and outputs interacting in the cycle (Fig. 1).
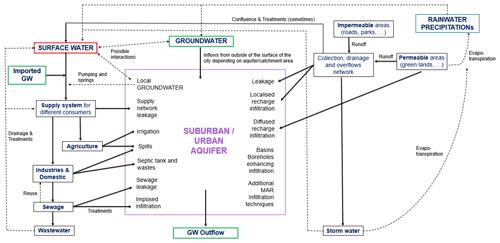
Figure 1Schematic view of the urban water cycle (modified from Wei et al., 2018; Barret et al., 1999).
The suburban context is more challenging because it presents many different pollution sources and contaminants (Fig. 2): the ones typical of an urban context, the ones which characterize the industrial activities and the ones linked to agricultural practice.
Having in mind that complexity in terms of contamination, it is unrealistic to talk about ideal conservative tracer unique to a specific source and pathway. The same substance in fact could have many different origins (the nitrate, for example, could be from agricultural practices and uses and from human contamination). Therefore, nowadays has been proven the better efficiency of using a multi-component and multi-tracer approach to distinguish between mixtures of pollutants.
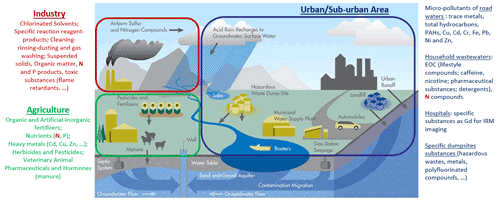
Figure 2Schematic synthesis of sub/urban contamination: pollutants and sources (modified by Peterson et al., 2007, from the link https://www.mrgscience.com/ess-topic-44-water-pollution.html, last access: 18 October 2022).
The first pilot site chosen for the project is a case of set of 4 abstraction wells located in Boussu, in the western Wallonia, in Belgium. The wells pump water from a semi-confined chalk aquifer: partly unconfined when it is outcropping, and partly covered by alluvial deposits and sandy-clay layers. The groundwater is flowing from S/SE to N/NW in the investigated portion of the chalk aquifer, and, on the basis of that observation and the historical data available on the site, a list of possible contamination sources is made (Fig. 3a). The network of monitoring wells and surface waters to be checked and studied in qualitative and quantitative terms, is determined afterwards, in function of the suspected contaminations and the potential pollution sources' locations. Investigations are limited to the so-called CAA (Catchment Area of the Abstraction) corresponding to the perimeter of the surface in which abstracted groundwater is recharged, and in particular to the PAFA (the Portion/Part of Aquifer Feeding the Abstraction) given the fact that the extensions were only linked to some safety reasons concerning the nature of surface waters flowing in the site. Those areas are already much larger than actual determined prevention zones (Fig. 3b).
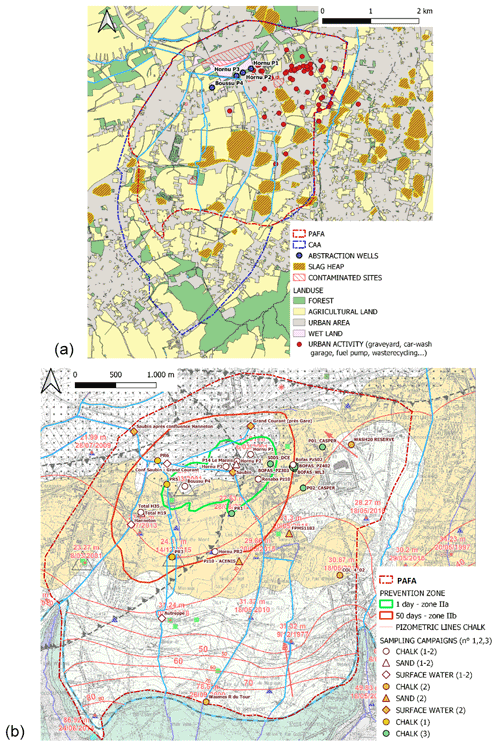
Figure 3(a) Map of all potential contamination sources present in the investigated site (© WalOnMap), (b) Monitoring network of sampling points for the three different campaigns performed, prevention zones and chalk aquifer pizometry (background map: Carte Quiévrain – Saint-Ghislain_4556).
Three different sampling campaigns were planned for a semi-circular panel of investigation in the W-S-E sides of the sites, in both aquifers, the chalk (points represented by circles in Fig. 3b) and the upper sand aquifer (triangle-shape points), and also in the surrounding surface water which might be infiltrating in the area (square-shape). Every time the surveys were more focused (and displaced) on the most impacted areas and sides of the site.
In the map it is possible to notice how the 1st and 2nd campaigns were more extended in the site, and samples were taken from surroundings surface waters and both chalk and sand aquifer. While the 3rd campaign mainly concentrates on the south-east side of the site, and only investigates the chalk aquifer.
The analyses performed are:
-
Physical parameters (ph, redox potential, dissolved O2, electrical conductivity and temperature);
-
Major elements (including Ca, Mg, Na, K, SO, NO, carbonate and bicarbonate ions (TAC), Cl, and also total and dissolved Fe and Mn);
-
Industrial pollutants, such as heavy metals, BTEXS, petroleum hydrocarbons EC5–EC11, petroleum hydrocarbons EC10–EC40, PAHs, halogenated aliphatic hydrocarbons, phenol, free cyanides and MTBE index;
-
Nitrogen forms (nitrate, nitrite, ammonium, Kjeldhal nitrogen TKN);
-
Organic matter content (TOC-total organic carbon, and COD-dissolved organic carbon);
-
Isotopes of nitrate, boron, sulphate and chlorinated solvents (these lasts only in the 3rd campaign);
-
Microbiological analyses based on molecular taxonomy (linked to the 16S RNA structure);
-
Specific markers as pharmaceutical substances (of different categories on the base of their use: human consumption, human medicines, agricultural substances, and animal health), and Gadolinium Gd (only during the 3rd campaign).
For almost every point, all analyses were performed (exception in very specific case where industrial pollutants presence was not an issue). The piezometers are sampled using a submersible pump according to the following protocols:
-
Measurement of the piezometric level;
-
Piezometer flushing: at least three times the volume of water contained in the piezometer or according to the 250 L standard volume or even until stabilisation of the in-situ physical parameters measured with a multi-parameter probe immerged in a continuous flow cell;
-
Measurement of in-situ parameters and collection of water samples according to the different requests and procedures (before filling, the non-pre-prepacked bottles are rinsed with water from the sampling point and filled to the top unless other indications);
-
Storage of samples in a dark place and in refrigerated coolers with ice packs before and during transport, and in a refrigerator and/or freezer until delivery to the various laboratories.
When the piezometers/wells are already equipped with pumps, water samples are taken from the tap. Surface water samples are taken using a peristaltic pump.
Table 1 summarises the details of the procedures (filtration, preservative and conservation, type of bottle) for the analyses chosen and indicates, for each, the laboratory in charge.
Stable isotopes of nitrate and boron are done to mainly distinguish urban effluents from agricultural fertilisers and manure (Nikolenko et al., 2018; Widory et al., 2005). Stable isotopes of sulphate are analysed to distinguish anthropic activity, sulphide minerals oxidation, sulphur-carbon compounds mineralisation, lixiviation, dissolution of evaporates, or alteration of carbon mines waste (Knöller et al., 2011). Those results are coupled with the occurrence of pharmaceutical (carbamazepine, etc.) and lifestyle (caffeine, nicotine) substances to identify anthropogenic contamination (Neufcourt, 2017) or agricultural pesticides-products and manure contamination substances; and also, with the occurrence of Gd (REE) which is used as a contrast agent in magnetic-resonance imaging, therefore it is suitable to verify contamination by anthropic wastewater and hospitals (Boester and Rüde, 2020; Petelet-Giraud et al., 2009). Furthermore, isotopes of chlorinated solvents are studied to understand if natural attenuation/degradation and/or other biochemical reactions are happening, and finally try to get closer to the location of their point source/origin (Åkesson et al., 2021). Microbiological and bacteriological analysis are also undertaken to make an inventory of all the microbial/bacterial species present in the samples, identifying populations associated with specific sources of pollution and verifying hypothesis on the occurrence of specific biochemical reactions (under peculiar conditions) in soil and groundwater, such as denitrification, sulphur reduction/oxidation, chlorinated solvents degradation, etc. (Kanohin et al., 2018; Krumar et al., 2014). Thus, first links between the abundance of different bacterial species and their affinity/resistance to some minerals/substances/conditions could be studied. The methodology used for the microbiological analysis is based on molecular taxonomy (linked to the 16S RNA structure). The procedure's steps are: (1) extraction of the total DNA on a filter; (2) amplification of the genetic sequences that constitute the intended target, (3) identification of the bacteria present on the basis of existing libraries, (4) identification of the most similar samples by nonmetric dimensional scaling.
Interpretation using multivariate methods and clustering (e.g.: SOMs, t-SNE, PCA, …) will be done later. The aims would be (1) to confirm some hypothesis already elaborated looking at the spatial distribution of measured concentrations of pollutants and the localisation of different contamination sources, and (2) the classification/subdivision of the sampled points in groups linked to their behaviour in the subsoil and the land use.
The main pollutants impacting the quality of the groundwater abstracted are: NO3, SO4 and Chlorinated Solvents. For each one of these pollutants, some specific analyses are performed in order to try to be able to differentiate their origins.
To briefly show some of the first results concerning the analysis cited above, following there are few graphs related to the 2021 summer sampling campaign (no. 2), which, when compared to the results obtained in autumn 2020 (campaign no. 1), show very similar trends, both in terms of major elements, specific substances occurrence and isotopes quantification. Figure 4 is showing the isotopes of nitrate and boron, as a function of NO3 concentrations (mg L−1). Groundwater chalk's samples are located among the manure, agricultural contamination (non-organic fertilisers) and atmospheric deposition boxes, which confirms that the nature of their nitrate concentrations is rather linked to agricultural origin. While, the surface water points fall into the wastewater box, which confirms their nature as sewers. Finally, there is only one point, corresponding to one of the abstraction wells, which is shifted to the right and that indicates possible denitrification (which might be confirmed by the increasing presence of nitrite and manganese and decreasing content of dissolved oxygen).
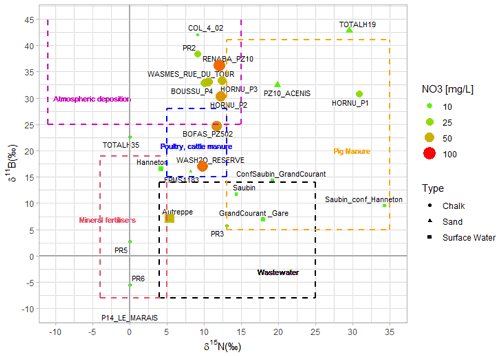
Figure 4Stable isotopes of nitrate (δ15N with a precision of ±0.4 ‰) and boron (δ11B ±2.6 ‰): results of the Boussu site for Summer 2021 (very similar results obtained in Autumn 2020).
Sulphate stable isotopes results (Fig. 5) show that the majority of the groundwater samples related to chalk aquifer are located between the boxes of sulphide oxidation, the presence of slag-heaps (located all around the site, especially in the SE side, where the chalk is outcropping) and the mineralisation of carbon and sulphur in the soil. The points that are most excluded are those of surface water and sand aquifer directly linked to the landfill present in the site: in fact they show a rather anthropogenic origin of SO4 contamination (mainly from the residential area east located). Finally, there are no obvious trends of bacterial sulphate reduction at this stage.
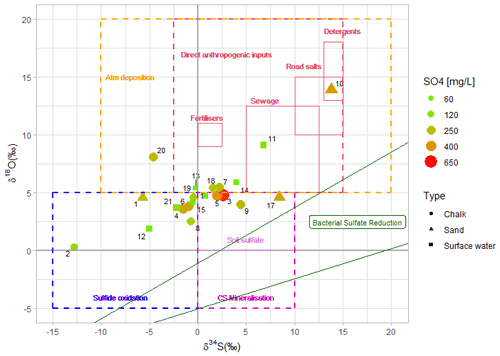
Figure 5Stable isotopes of sulphate (δ34S ±0.4 ‰ and δ18O ±0.6 ‰): results of the Boussu site for Summer 2021(the first campign in which those analysis where tested).
The pharmaceutical substances are also analysed: a selection of few substances for each of the 4 categories analysed using their occurrence mainly as a proof of hypothesis contamination's origin (Fig. 6). Those results will be also coupled with Gd occurrence and anomalies, once the data will be available.
There are no remarkable trends shown by the concentrations and spatial distributions, but few observations are pointed out:
-
The 4 pumping wells together with the closer piezometers do not have any “life-style” substances.
-
All samples contain substances related to human health (possibly linked to the presence of an open sewage system via canals flowing in the studied site) and to agricultural activity (justified by the fields surrounding the south/south-east side of the site). This as a proof of the mixed context investigated.
-
the dichlorobenzamide is present almost everywhere: this substance is the main metabolite of the herbicide “dichlobenil” which was used on a large scale also in non-agricultural areas and in cemeteries until it was banned in 2013.
-
The occurrence of pharmaceuticals for human health is detected especially in correspondence with the chalk wells, in trace amounts in the two sand samples, and in much larger quantities in the surface waters. The drugs related to human health found in the pumping wells (carbamazepine, hydrochlorothiazide, fenofibric acid) are not necessarily the same as those found in the samples located south-east (Paracetamol, Sotalol).
-
The unique and very low concentrations of animal drugs are found in the well corresponding to the landfill.
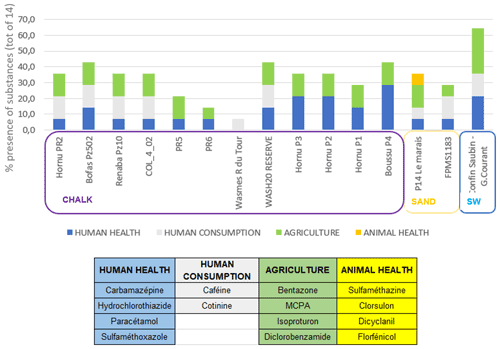
Figure 6Occurrence of pharmaceutical substances in the sampling points of Boussu site (coupling the results from Autumn 2020 and Summer 2021): the table on the right summarizes the selection of substances for each category (maximum of 4), on the base of results on detected pharmaceuticals in the site and following the results of two other projects on that topic, IMHOTEP (Nott et al., 2018); BIODIEN (Frippiat et al., 2018).
Chlorinated solvents are mainly found in the chalk aquifer samples, with the highest concentrations measured in the east side of the site. Therefore, the source of that pollution seems to be located to the east, very close to a new hospital/ancient fuel station. The spring 2022 campaign shows a focus on the wells located closer to the area where the source is supposed to be and where possibly some degradation reactions are happening. To go further on this interpretation, analysis on gradient concentrations, molar ratio, plume behaviour and isotopes of Carbon, Hydrogen and Chloride will be performed, together with more research of historical and possibly former pollutants activities impacting the site.
Data from the 3rd sampling campaign (spring 2022) are still not available. Microbiological analysis results are also not showed in this manuscript, because interpretation are still going on.
The approach illustrated is a rigorous methodology to assess pollution sources. The analysis and interpretation done until now are just part of the investigations which are still going on.
Clustering and multivariate analysis will be performed on the chalk aquifer, to prove some of the previous reflexions and while collecting more information on how to group and categorized the points of the area while looking at the collected data.
A hydrogeological model will be optimized to simulate present/future contamination from the known/discovered sources of pollution.
Finally, to confirm the hypotheses, especially on the origin of sulphates and chlorinated solvents, additional field investigations must be carried on: an expansion of the sampling network at the level of the chalk is planned (drilling of new piezometers, use of additional wells in the proximity of the detected possible sources) together with the intention to measures the groundwater fluxes via FVPDM technique (Brouyère et al., 2018). The fluxes measures will also help in the hydrogeological model calibration and optimization and will be coupled with hydrogeochemical results.
As last, the development of useful tools to prioritize investigation and remediation measures should be generalized from that specific case to a more general one, in order to try to have a referential procedure to follow upon qualitative and quantitative studies in an aquifer.
Data are not publicly accessible, but further information about the data can be obtained directly from the authors.
All authors conceived of the presented idea. LB aided in gathering and processing the data. All authors provided critical feedback and helped shape the research-analysis. All authors discussed the results and commented on the manuscript.
The contact author has declared that none of the authors has any competing interests.
Publisher's note: Copernicus Publications remains neutral with regard to jurisdictional claims in published maps and institutional affiliations.
This article is part of the special issue “Quality and quantity issues in urban hydrogeology (EGU2022 HS8.2.8 session)”. It is a result of the EGU General Assembly 2022, Vienna, Austria, 23–27 May 2022.
The authors thank to SPGE (Société Publique de Gestion de l'Eau) to finance the project. The authors also express their gratitude to SWDE (Société Wallonne Des Eaux), SPAQuE, the research unit of Aquapôle-ULiège and FMV-ULiège for their support, aid and active participation during the study. We appreciate the constructive comments from the reviewers.
This paper was edited by Estanislao Pujades and reviewed by Diego Schmidlin and one anonymous referee.
Åkesson, S., Sparrenbom, C. J., Paul, C. J., Jansson, R., and Holmstrand, H.: Characterizing natural degradation of tetrachloroethene (PCE) using a multidisciplinary approach, Ambio, 50, 1074–1088, https://doi.org/10.1007/s13280-020-01418-5, 2021.
Barrett, M. H., Hiscock, K. M., Pedley, S., Lerner, D. N., Tellam, J. H., and French, M. J.: Marker species for identifying urban groundwater recharge sources: A review and case study in Nottingham, UK, Water Res., 33, 3083–3097, https://doi.org/10.1016/S0043-1354(99)00021-4, 1999.
Boester, U. and Rüde, T. R.: Utilize gadolinium as environmental tracer for surface water-groundwater interaction in Karst, J. Contam. Hydrol., 235, 103710, https://doi.org/10.1016/j.jconhyd.2020.103710, 2020.
Brouyère, S., Jamin, P., Orban, P., Dassargues, A., and Cosme, F.: Advanced single-well applied tracer techniques for improving reliability of groundwater and contaminant mass flux monitoring. Paper presented at Conference on Remediation of Chlorinated and Recalcitrant Compounds, April2018, Palm Springs, CA, https://orbi.uliege.be/bitstream/2268/222316/2/D6_1210_%23422_Brouy%C3%A8re.pdf (last access: 15 September 2022), 2018.
Frippiat, C., Bémelmans, S., Burlion, N., Carbonnelle, P., Chalon, C., Delvaux, A., Galloy, A., Marneffe, Y., Nadin, C., Nix, Ph., Nott, K., Pigeon, O., Ronkart, S., Rousseau, G., Delloye, F., and Brahy, V.: Recherche de perturbateurs endocriniens et d'autres substances d'intérêt récent dans les eaux en vue de la protection de la santé publique et de l'environnement, Programme de Recherche “BIODIEN – Rapport final, GISREAUX, rapport no. 2018-01690, 199 p. + 11 annexes, http://eau.wallonie.be/IMG/pdf/2018-01690_GISREAUX_BIODIEN_Final_%20Rapport.pdf (last access: 10 October 2022), 2018.
Kanohin, F., Otchoumou, E., Yapo, O. B., Dibi, B., and Bonny, A. C.: Caractérisation physico-chimique et bactériologique des eaux souterraines de Bingerville, Int. J. Biol. Chem. Sci., 11, 2495–2509, https://doi.org/10.4314/ijbcs.v11i5.43, 2018.
Knöller, K., Trettin, R., and Strauch, G.: Sulphur cycling in the drinking water catchment area of Torgau–Mockritz (Germany): insights from hydrochemical and stable isotope investigations, Hydrol. Process., 19, 3445–3465, https://doi.org/10.1002/hyp.5980, 2005.
Krumar, A., Nirpen, L., Ranjan, A., Gulati, K., Thakur, S., and Jindal, T.: Assessment of chemical and microbial contamination in groundwater through leaching of sewage waste in Delhi, India, ASIAN J. Environ. Sci., 9, 37–48, https://doi.org/10.1007/s12665-015-5016-0, 2014.
Neufcourt, G.: Utilisation de substances médicamenteuses comme traceurs du nitrate provenant des eaux usées par opposition à l'origine agricole, Master thesis/Mémoire de fin d'études, Université de Liège, Faculté des sciences appliquées, Département ArGEnCo, Liège, 109 pp., 2017..
Nikolenko, O., Jurado, A., Borges, A. V., Knöller, K., and Brouyère, S.: Isotopic composition of nitrogen species in groundwater under agricultural areas: A review, Sci. Total Environ., 621, 1415–1432, https://doi.org/10.1016/j.scitotenv.2017.10.086, 2018.
Nott, K., Gillet, M., Carbonnelle, P., Frippiat, C., Moutier, M., Ronkart, S., Delloye, F., and Brahy, V.: Recherche des substances émergentes dans les eaux et intéressant la santé publique et l'environnement, Programme de Recherche “IMHOTEP” (Inventaire des Matières Hormonales et Organiques en Traces dans les Eaux Patrimoniales et Potabilisables) – Rapport final, 409 pp. + 6 annexes, http://etat.environnement.wallonie.be/files/Studies/2018_IMHOTEP.pdf (last access: 10 August 2022), 2018.
Petelet-Giraud, E., Klaver, G., and Negrel, P.: Natural versus anthropogenic sources in the surface- and groundwater dissolved load of the Dommel river (Meuse basin): Constraints by boron and strontium isotopes and gadolinium anomaly, J. Hydrol., 369, 336–349, https://doi.org/10.1016/j.jhydrol.2009.02.029, 2009.
Peterson, J. L., McFarland, M. L., Dictson, N., Boellstorff, D., and Berg, M.: Texas Watershed Steward Handbook: A Water Resource Training Curriculum., Texas AgriLife Extension Service, The Texas A&M University System – Department of Soil and Crop Sciences, College Station, TX, 138 pp., 2007.
Wei, H., Wang, Y., and Wang, M.: Characteristic and pattern of urban water cycle: Theory, Desalination and Water Treatment, 110, 349–354, https://doi.org/10.5004/dwt.2018.22342, 2018.
Widory, D., Petelet-Giraud, E., Négrel, P., and Ladouche, B.: Tracking the Sources of Nitrate in Groundwater Using Coupled Nitrogen and Boron Isotopes: A Synthesis, Environ. Sci. Technol., 39, 539–548, https://doi.org/10.1021/es0493897, 2005.