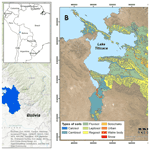
Insights on the hydrological cycle and its interaction with anthropic pressures: the Katari basin and minor Titicaca lake
Rotman A. Criollo Manjarrez
Laura Scheiber
Laura Poza
Sonia Valdivielso Mijangos
Pedro Simunovic
Enric Vázquez-Suñé
The hydrologic cycle behaviour in the Bolivian Altiplano and how it interacts with human activity are still not fully understood. The hydraulic cycle is examined in a novel way in this work by considering extended time series of associated hydrologic and climatic data. Results show that human exploitation and losses from the sewage and water supply networks in the urban areas might cause an hydraulic imbalance in the region. These anthropogenic incomes, at the same time, increase concentrations of different pollutants. Water isotopic analysis reinforces the conceptual model showing that the groundwater sampled are mainly from precipitation. Workshops with local municipalities supported and defined the most relevant anthropic pressures in the basin. This information supports a vulnerability analysis in collaboration among them. The decision-making process for government entities might be strengthened by this study, which would help to develop long-term strategies to prevent and mitigate the issues found in the evaluation of water vulnerability in the region.
- Article
(6387 KB) - Full-text XML
- BibTeX
- EndNote
Arid and semi-arid ecosystems are highly sensitive and vulnerable, particularly in the current climate change context. These environments support traditional local human populations and other beneficial human activities (i.e., human water supply, agriculture, raising animals, urbanization, mining, industry and tourism) (Valdivielso et al., 2022). In that water scarce regions, groundwater is one of the main water resources and Bolivia is not an exception. Approximately 10 % of the Bolivian population (one million inhabitants) lives in the Katari basin (INE, 2022). It is one of the most populated area which leads to an increase of anthropic pressures (e.g., increase on water and energy demand, diffuse pollution sources, agricultural activities, abandoned local mining, urban water and solid waste discharges). This in turn affects the quantity and quality of both surface and underground water resources available.
Few studies have been performed in the area aiming to improve on the environmental protection and water resources (Alanoca, 2018; CEPAL, 2000; CONIAG/CGIAB, 2002; COIPASA, 2014; IDB, 2013, 2018; MMAyA, 2010, 2014a, b, c, 2015a, b, 2016, 2018; UNEP, 1996), to understand the geometry of the subsurface basin such as geology at deep depths or the boundaries and thickness of the aquifers (Flores Avilés et al., 2020), and the state and the evolution of the groundwater quality (Quino et al., 2019; Salvarredy-Aranguren et al., 2008; Paz, 2009). Despite these advances, the general picture of the hydraulic cycle and its interaction with anthropic pressures of the area are not widely understood.
The purpose of this paper is to provide a quantitative framework of the hydraulic cycle for improving sustainable water management and assessing the environmental risks and/or anthropic impacts in the basin. After the validation of historical data compiled and field campaign measurements (2019), the conceptual model developed serve as an input to discuss together with different municipalities impacts and pressures in the basin in order to create a vulnerability analysis.
2.1 Study area
The Katari basin is located in the Andean region known as the Altiplano, between 3800 and 5200 m above sea level (m a.s.l.) and it has an extension of 4280.5 km2. It is a foreland basin located between the Eastern and Western Cordillera of the Andes. Climatic variations have been the main causes of the evolution of the Altiplano. Lakes have grown as a result of the alternating of wet and dry, warm and glacial episodes. These elements correlate to lacustrine formations in the centre of the Altiplano, river sediments in the foothills and plains, and glacial deposits (moraines) in the nearby mountain ranges. (Fig. 1). The lake surface was 200 m above current sea level at the start of the Pleistocene, covering more than 50 000 km2. In other words, it covered the entire Altiplano. In the late Pleistocene successive glacial cycles took place that caused the progressive reduction of the lacustrine surface. This reduction produced the water sheet of Lake Titicaca to reach lower levels than the current ones, up to 60 m below the current sea level. Lake levels fluctuated throughout the Holocene, reaching up to 30 m in some cases. Rivers that flowed into Lake Titicaca eroded beds and formed channels that extended several hundred meters into the current lake during this time period.
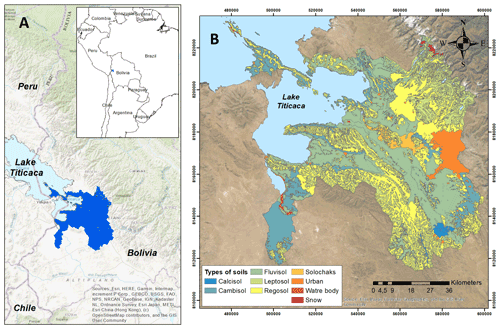
Figure 1Katari basin (Bolivia), topographic basemap from ArcGIS (ESRI). Hydrogeological units (a) considered according with Alanoca (2018): UH1 (yellow), UH2 (orange), UH3 (pink), and UH4 (green) Types of soils (b) classified by FAO (MMAyA, 2018).
At present, the geological configuration of the Altiplano is as follows: The NE part of the basin (Cordillera Oriental) is composed of Silurian and Devonian sandstones, shales, siltstones and slates. In the Huayna Potosí mountain, igneous rocks from the Triassic outcrop. To the south of the basin, in the interaltiplanic mountain ranges, Devonian sandstones, shales, siltstones and slates outcrop. In the lower part of the basin, fluvio-lacustrine deposits are predominant below the terraces of the former Ballivian Lake (Coudrian-Ribstein et al., 1995). Alluvial and fluvio-glacial deposits made the UH1 unit, while alluvium and discontinuous fluvial deposits produce UH2 (generally located in lower areas of the main riverbeds) (Fig. 1).
2.2 Sample collection
Field campaign performed on September 2019 update information compiled from different sources described in the introduction section. During this campaign hydrogeological, physical and chemical measures were obtained following the methodologies described hereafter.
Groundwater samples were collected from a total of 13 piezometers (5–60 m deep), distributed throughout the study area. Wells were purged and samples collected after removing three well volumes or once field parameters had stabilized. The physico-chemical parameters such as temperature (∘C), pH, Specific Conductance (SC, µS cm−1), Eh and dissolved oxygen (DO, mg L−1) were measured in situ inside a closed flow cell. Total alkalinity was determined in the field by acid-base titration using an Aquamerck Alkalinity kit.
Groundwater samples for common chemistry were collected, previously filtered through a 0.22 µm nylon filter, in high-density polyethylene (25 mL bottles for anions and 50 mL for cation-trace samples). Cation-tracer samples were acidified with 1 mL of 20 % diluted nitric acid for sample preservation. Anions were analysed by High Performance Liquid Chromatography (HPLC) and cations by Inductively Coupled Plasma Atomic Emission Spectrometry (ICP-AES). Inductively coupled plasma and mass spectrometry (ICP-MS) was used to estimate concentrations of the trace elements. Field speciation cartridges were used to differentiate Arsenite (As (III)) from Arsenate (As(V)). 30 mL glass bottles previously muffled was used to collect samples to measure Dissolved Organic Carbon (DOC). These samples were filtered through a 0.45 µm and acidified with 1 mL of HCl (2N). Bottles were sealed with Parafilm® to minimize any contact with air. DOC was analysed by the catalytic oxidation method at 680 ∘C using a Shimadzu TOC-V CSH instrument, with a detection limit of 0.05 mg L−1. NH and I− concentrations were analysed with ion selective electrodes Orion 9512, with an error of ±2 %.
2.3 Data management
Multiple sources listed in the introduction section were used to gather data on the hydrological cycle. Most of this information were generated and managed by the Katari Basin Management Unit (UGCK) and the Ministry of the Environment (MMAyA), Bolivia.
These datasets were checked for accuracy, made homogeneous, migrated, and integrated using GIS-based tools (Velasco et al., 2013). This GIS-based platform facilitates and improves the reporting of groundwater quality and quantity results. Aditionally, this platform is connected with external software to perform further analyses: hydrochemical balance (Easy_Quim, Serrano-Juan et al., 2020), water mix (MIX, Carrera et al., 2004), and interpretation of hydraulic parameters (MJ-Pumpit, Criollo et al., 2016).
2.4 Hydraulic balance
The hydraulic balance was calculated using the balance of water content in the soil from 20 years of intermittent data: determination of the spatial and temporal distribution of precipitation and temperature in the study area; evaluation of the runoff factor and the lamination value through hydrogeological analysis, among others; determination of the soil properties (types of soil, spatial distribution, root thickness, etc.) based on FAO standards (Fig. 1b) and soil physiology; calculation of the potential and real evapotranspiration; and the balance of water in the soil. The United States Secretary of Agriculture (USDA) classification was used to estimate the textures of these soils (Schroeder et al., 1994).
The Potential Evapotranspiration (ETP) and recharge were estimated using EASY_BAL (Serrano-Juan et al., 2020). This software estimates the aquifer recharge from precipitation and irrigation. It includes the Thornthwaite method (Custodio and Llamas, 1996) to estimate the monthly ETP. Then, based on the amount of precipitation accumulated during the time period under consideration as well as the features of the soil in terms of its water availability, the real evapotranspiration (ETR) is calculated. Finally, a water surplus or deficit that will represent the aquifer's recharge is predicted using the ETR data.
Aquifer recharge in urban areas was estimated using the method proposed by Tubau et al. (2017) considering urban runoff, sewage and water supply networks.
The Darcy equation has been used to determine the outflow from the study area to the Minor Lake of Titicaca. The perimeter of the lake was separated into various portions based on the hydrogeological parameters and piezometry (Fig. 2). The groundwater outflows produced by the pumping were calculated according to the data provided by the Katari Basin Management Unit (UGCK). For the entire study area, flows have been obtained from 42 wells belonging to the Empresa Publica Social de Agua y Saneamiento (EPSAS).
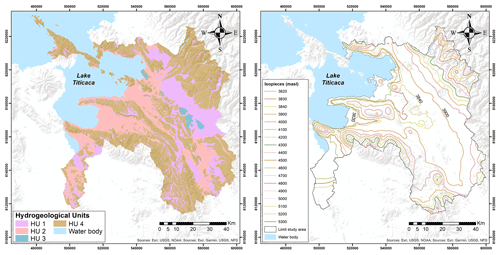
Figure 2Piezometric map (a) obtained using data compiled (scale 1:10 000, basemap source: ArcGIS, ESRI). Transmissivity map of the hydrogeological units interpreted according with Alanoca (2018) (b).
Wetlands are fundamental ecological functions (source of surface water and habitat of rich biodiversity) and embrace wetlands (green areas) and minor lagoons. They constitute groundwater discharge areas calculated as real evapotranspiration (ETR) in the case of wetlands and evaporation of free water sheet (EV) in minor lagoons. As in the previous cases, the calculation was performed applying EASY_BAL program.
Due to the information available, the transmissivity values were estimated using the Galofré method (Eq. 1, Custodio and Llamas, 1996).
This allowed to obtain reasonable transmissivity values in 98 of the 811 registered water points. Because their spatial distribution is limited and does not cover the entire study area, bibliographic data is required (Alanoca, 2018) and the classification of hydrogeological units, mentioned in previous sections, were used to cover this information.
2.5 Vulnerability analysis
The vulnerability analysis of the aquifers allows a diagnosis of the basin and identified problems/pressures that are considered by the actors in charge of managing water resources. A workshop was performed on September, 2019 at the Katari Basin Management Unit (UGCK) headquarters and together with the participation of different municipalities: Laja, Pucarani, Viacha, Tihuanacu and Desaguadero. The major goals of the workshop were to identify and evaluate the anthropic pressures that have an influence on the water resources, identify corrective measures that are being used, and define corrective measures that might be applied. The DRASTIC method (Aller et al., 1987) was used and then modified including the information obtained from this workshop to create vulnerability maps for the improvement of the water resources management.
3.1 Hydrogeology
The conceptual model of the Katari basin consider natural and anthropic inflows and outflows in the system. Annual precipitation in the area is 624.57 hm3 yr−1. Transmissivities of the hydrogeological units (Fig. 2a) compiled and calculated are consistent with Flores Avilés et al. (2020). The recharge from snow and glaciers was calculated using Liu et al. (2016) runoff values, yielding an aquifer recharge of 17.1–14.5 hm3 yr−1.
The piezometric map (Fig. 2b) shows the direction flow through isolines. In the places with higher topographic relief, groundwater is replenished (i.e., precipitation of rain and melting ice). This water flows into the centre, lowest region of the basin, where the majority of rivers serve as effluents. With the exception of waters from the Desaguadero basin, which flow into the PooPó Lake (topographically lower, outside the boundary of the study area), the Katari Basin empties into Cohana Bay (Minor Lake) and into wetlands (bofedales).
Aquifer recharge in urban areas occurs mainly by leakages in the supply and/or sewage networks (Tubau et al., 2016). According with MMAyA studies (MMAyA, 2018), water sewage leakages is directly related to the water supply with an estimation of 80 %. In other hand, urban surface runoff can be calculated as the proportion of precipitation (runoff coefficient) that enters the sewage system during rain events. Applying a runoff coefficient of 70 % and estimated losses of the sewage network around 5 %, a total recharge of 5.9 hm3 yr−1 was calculated.
The outflow from the study area to Lake Titicaca has been calculated following the Darcy equation (Custodio and Llamas, 1996). It was applied for eleven boundaries of different hydrogeological units at the boundary with the Titicaca Lake obtaining a 129.72 hm3 yr−1 of discharge.
The discharge flow to the rivers is also influenced by the base flow value considered. In this way, for a base flow of 29 % and 105, a discharge of 166.75 and 57.5 hm3 yr−1 is obtained respectively.
Water discharge from wetlands were assumed for a wet surface of approximately 240 km2, resulting in a total ETR of 99.38 hm3 yr−1. On the other hand, minor lagoons, or small water masses, in the region are thought of as a sheet of water free of water (total area estimated at about 23.03 km2), leading to an evaporation of 13.45 hm3 yr−1.
Finally, extraction of the aquifers was obtained from 42 wells. To cover the information gaps and ensure that the withdrawal data is the most representative of the study area, water withdrawals from the 2008–2017 period were considered estimating this outflow as 10.74 hm3 yr−1.
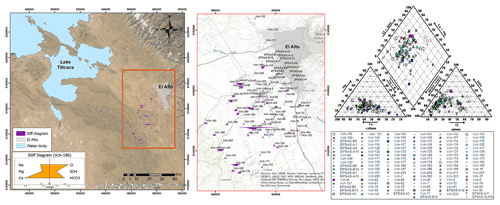
Figure 3Hydrochemical data compiled (1987–2018) including latest campaign (2019). The mean compositions of the data gathered at each point are shown in the left and middle figures (Stiff diagram). Wide variations between bicarbonate-calcium and bicarbonate-sodium waters are shown in the right picture (Piper diagram) (right figure). There are significant differences in Ingavi's center and boundaries (areas with an increase industrial development). Source for basemap: ArcGIS (ESRI).
3.2 Hydrochemistry
As in the case of piezometric information, spatial distribution of quality data compiled and collected is heterogenous, especially closest to the Lake Titicaca. Figure 3 show wide range of water types using hydrochemical diagrams. Most of the waters are calcium-bicarbonated (16 % of the samples collected in the 2019 campaign) and sodium-bicarbonated (66 % of the samples collected in the 2019 campaign). Thus, it can be said that the predominant anion in groundwater is bicarbonated (95 %) and the main cations are calcium and sodium. In minor percentage samples with a sodium-chloride composition are observed (18 % of the samples obtained in the 2019 campaign), these are located in the vicinity of the Seco River, the Colorado River. These values agree with Flores Avilés et al. (2022) findings for samples collected in altitudes ranging from 3860 to 4600 m a.s.l. at the north eastern of the study area.
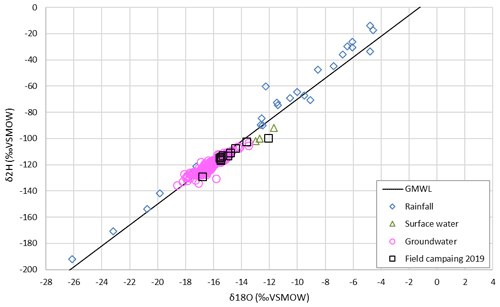
Figure 4Isotopic values of the water: rainfall, surface water, groundwater samples compiled and groundwater samples from the field campaign performed during 2019. Most of these samples align with SMOW line.
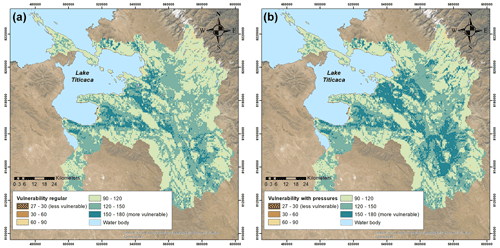
Figure 5Vulnerability results considering common DRASTIC method (a) and considering anthropic pressures (b).
In general, concentrations of major and trace ions in the sampled waters do not present anomalous values, except for some specific cases. As a reference, the limits established by the World Health Organization (WHO, 2017) criteria for the quality of water for human consumption have been taken. It was detected that some points show excess WHO standards: Chlorides (250 mg L−1), Sulfates (250 mg L−1), Nitrates (50 mg L−1), Manganese (0.4 mg L−1), Arsenic (0.1 mg L−1), Lead (0.1 mg L−1) and Boron (0.5 mg L−1). The presence of these concentrations seems to be related to the interaction of groundwater with the mineralization of the Eastern Andes Tin Belt (Faja Estañífera de los Andes Orientales), which enters the eastern part of the Lake Titicaca basin. To the west of the Tin Belt is the Plumbo – Silver Belt, adjacent to Lake Titicaca, while to the southeast of the lake lies the copper belt. These results agree with works performed by Paz (2019) where is showed that the groundwater of the Katari basin has heavy metals such as Fe, Mn, Cu, Cd, Zn and Pb. Following this behavior, it is observed that 42 % of the sampled points have Arsenic concentrations that exceed the permitted limit for drinking water set by the WHO, while 50 % of the sampled points have Lead concentrations that exceed the permitted limit for drinking water set by the WHO. Samples with chloride values higher than 250 mg L−1 and Manganese values higher than 400 ppb (0.4 mg L−1) are located near the Pallina river between the municipalities of Laja and Viacha and in the south of Viacha (Fig. 3). Sulfates concentrations follow a similar pattern, with the highest values found between the towns of Laja and Viacha. However, there is also a spot with a high concentration of sulphate that is found north of Alto (Fig. 3). The latest location also present concentrations of nitrates higher than 50 mg L−1, specifically near the Seco river and along Julio Cesar Valdez Cardona avenue. Finally, the samples with Boron values higher than 500 ppb (0.5 mg L−1) are located in the areas closest to Lake Titicaca.
The isotopic characteristics of water sampled (deuterium, δD, and oxygen, δ18O) provides information on the origin of groundwater from the possible processes that may have caused changes in the isotopic composition of water. Its content is expressed as a deviation in ‰ with respect to the Standard Mean Ocean Water point (SMOW). The content of δD and 18O is conditioned by: (i) climatic factors (temperature, evaporation, thermal processes, etc.); and (ii) geographical factors (continentality, altitude, latitude, etc.).
The relationship between δD and δ18O in the world mean meteoric water obeys the following relationship:
where d is the excess of deuterium, which is characteristic of the rain of each place and for this reason it can be used as an isotopic marker. δD values are between −129.15 ‰ and −99.73 ‰ (SMOW) and δ18O values range between −16.8 ‰ and −12.07 ‰ (SMOW). Figure 4 represents the relationship between δD and δ18O with respect to the global meteoric line. The samples collected during the 2019 field campaign generally show comparable values of compiled information of precipitation, surface water, and groundwater data. Additionally, because every sample is aligned with the global meteorological line, the majority of the water in the aquifer corresponds to precipitation, which replenishes the aquifers.
3.3 Vulnerability
The vulnerability analysis allows a diagnosis of the basin to be made and the problems that are considered a priority by the actors in charge of managing water resources to be identified.
The anthropic pressures in the environment was determined from the workshop performed together with stakeholders of the Katari basin. These information was included in the DRASTIC method to evaluate the vulnerability of the aquifers. Figure 5 shows the variations in vulnerability assessments that take into account or do not take into account the environmental expertise of the communities participating in the decision-making process for the water resources. Human activities that have the most effects on the water bodies of the Katari basin are intensive-extensive livestock farming, mining (extraction of clay mainly) and losses from septic tanks and the supply network. According to the hydraulic balance, these losses are essential to comprehend how the recharge system works. The SIGAR program (2014) evaluated 46 localities in the circumlacustrine ring of Lake Titicaca, 28 in Peru and 18 in Bolivia. They represent 90 % of all the discharged water. Only 30 have a Wastewater Treatment System from the 46 localities, while the other 16 use latrines and septic tanks producing leaching of contaminants until they reach the aquifer levels.
The current study supports previous research in this area. It would provide a reference framework for other studies or models of greater detail. The isotopic analysis confirmed that most of groundwater corresponds to precipitation and support the conceptual model. It shows the role of different natural and anthropic sources in the hydraulic balance of the aquifers. Nevertheless, it still includes uncertainties because of the spatial and temporal resolution of the datasets available. Although the fact that the water uses on rural communities have a minor impact on the hydraulic balance, it would be convenient to consider them in future studies.
Groundwater quality, in general, do not present anomalous values, except for some specific cases that exceed the permitted limit for drinking water set by the WHO. This quality feature is confirmed during the workshop with different stakeholders where the most relevant anthropic pressures were defined. This information, at the same time, improved the vulnerability analysis. This evidence shows that by incorporating their environmental knowledge, the global understanding of aquifer behaviour and susceptibility can be enhanced, which could boost cooperation between various parts. However, workshops with all municipalities would be required to improve the vulnerability analysis and engage collaborations among different stakeholders in the area.
Software used can be made available upon request to the corresponding author or it can be freely downloaded from https://www.idaea.csic.es/research-group/groundwater-and-hydrogeochemical/ (IDAEA-CSIC, 2022)
Data compiled is available from references described at Sect. 2.3 (data management). Data collected during the field campaign is available upon reasonable request to the corresponding authors.
All the authors compiled, validated and analysed data used in this work. RACM, SVM, LS and LP made figures. RACM wrote the manuscript with input from all the authors LS and EVS, in collaboration with UCGK and MMAyA, carried out the field campaigns and managed the workshop. LS, LP, SVM, PS and RACM created the vulnerability analysis. EVS coordinated the study. All authors discussed results and edited the paper.
The contact author has declared that none of the authors has any competing interests.
Publisher’s note: Copernicus Publications remains neutral with regard to jurisdictional claims in published maps and institutional affiliations.
This article is part of the special issue “Quality and quantity issues in urban hydrogeology (EGU2022 HS8.2.8 session)”. It is a result of the EGU General Assembly 2022, Vienna, Austria, 23–27 May 2022.
The authors thank to UGCK and MMAyA for their support and aid during the study. The authors also express their gratitude the local communities for their active participation in the workshop. We appreciate the constructive comments from the anonymous reviewers.
We acknowledge support of the publication fee by the CSIC Open Access Publication Support Initiative through its Unit of Information Resources for Research (URICI).
This paper was edited by Estanislao Pujades and reviewed by Laura Balzani and one anonymous referee.
Alanoca, D.: Evaluación de la recarga del sistema acuífero Purapurani de la cuenca Katari [Evaluation of the recharge of the Purapurani aquifer system of the Katari basin], Degree thesis, Universidad Mayor de San Andrés, Bolivia, http://repositorio.umsa.bo/xmlui/handle/123456789/20397 (last acess: 5 July 2022), 2018.
Aller, L., Bennett, T., Lehr, J. H., Petty, R. H., and Hackett, G.: DRASTIC: a standardized system for evaluating groundwater pollution potential using hydrogeologic settings, USEPA Report 600/2-87/035, Robert S. Kerr Environmental Research Laboratory, Ada, Oklahoma, 1987.
Autoridad binacional autonoma del sistema hidrico, lago Titicaca, rio Desaguadero, lago Poopó (COIPASA): Programa SIGAR, Sistema Integral de Gestión de Aguas Residuales, Diagnóstico y Categorización de los Sistemas de Tratamiento de Aguas Residuales en el anillo Circunlacustre del Lago Titicaca, Bolivia [Autonomous binational authority of the water system, Lake Titicaca, Desaguadero River, Lake Poopó (COIPASA): SIGAR program, Comprehensive Wastewater Management System, Diagnosis and Categorization of the Wastewater Treatment Systems in the Circumlacustrine Ring of Lake Titicaca. Bolivia], 2014.
Banco Interamericano de Desarrollo (IDB): Estado de conservación de los ecosistemas en el área de influencia del proyecto multipropósito de recursos hídricos para la zona metropolitana de la Paz y el Alto, [State of conservation of ecosystems in the area of influence of the multipurpose water resources project for the metropolitan area of La Paz and El Alto. Inter-American Development Bank (IDB)], 2013.
Banco Interamericano de Desarrollo (IDB): Apoyo a la Implementación del Plan Maestro de la Cuenca Katari del Lago Titicaca, BO-T1252. Banco Interamericano de Desarrollo (IDB), Bolivia [Support for the Implementation of the Master Plan for the Katari Basin of Lake Titicaca, BO-T1252, Inter-American Development Bank (IDB), Bolivia], 2018.
Carrera, J., Vázquez-Suñé, E., Castillo, O., and Sánchez-Vila, X.: A methodology to compute mixing ratios with uncertain end-members, Water Resour. Res., 40, W12101, https://doi.org/10.1029/2003WR002263, 2004.
Consejo Interinstitucional del Agua (CONIAG) y Comisión para la gestión integral del agua en Bolivia (CGIAB): Disponibilidad, uso y calidad de los recursos hídricos en Bolivia, Cumbre Mundial sobre el Desarrollo Sostenible, Johannesburgo [Availability, use and quality of water resources in Bolivia, Interinstitutional for Water (CONIAG) and Commission for the integral management of water in Bolivia (CGIAB), World Summit on Sustainable Development, Johannesburg], 2002.
Coudrain-Ribstein, A., Pratz, B., Quintanilla, J., Zuppi, G. M., and Cahuaya, D.: Salinity of the groundwater on the Central Altiplano 24, Bulletin de l' Institut Français d'Études Andines, 483–493, 1995.
Criollo, R., Velasco, V., Vázquez-Suñé, E., Serrano-Juan, A., Alcaraz, M., and García-Gil, A.: An integrated GIS-based tool for aquifer test analysis, Environ. Earth Sci., 75, 391, https://doi.org/10.1007/s12665-016-5292-3, 2016.
Custodio, E. and Llamas, M. R.: Hidrología subterránea, Editorial Omega, Barcelona, ISBN 9788428204477, 1996.
Flores Avilés, G. P., Descloitres, M., Duwig, C., Rossier, Y., Spadini, L., Legchenko, A., Soruco, A., Argollo, J., Pérez, M., and Medinaceli, W.: Insight into the Katari-Lago Menor Basin aquifer, Lake Titicaca-Bolivia, inferred from geophysical (TDEM), hydrogeological and geochemical data, J. South Am. Earth Sci., 99, 102479, https://doi.org/10.1016/j.jsames.2019.102479, 2020.
Flores Avilés, G. P., Spadini, L., Sacchi, E., Rossier, Y., Savarino, J., Ramos, O. E., and Duwing, C.: Hydrogeochemical and nitrate isotopic evolution of a semiarid mountainous basin aquifer of glacial-fuvial and paleolacustrine origin (Lake Titicaca, Bolivia): the efects of natural processes and anthropogenic activities, Hydrogeol. J., 30, 181–201, https://doi.org/10.1007/s10040-021-02434-9, 2022.
IDAEA-CSIC: Groundwater and Hydrogeochemistry, https://www.idaea.csic.es/research-group/groundwater-and-hydrogeochemical/, last access: 9 November 2022.
Instituto Nacional de Estadística (INE): https://www.ine.gob.bo/index.php/prensa/notas-de-prensa/item/2317-viacha-lidera-en-la-produccion-de-cebada-en-grano-en-el-departamento-de-la-paz, last access: 13 August 2022.
Liu, T., Kinouchi, T., Mendoza, J., and Iwami, Y.: Glacier mass balance and catchment-scale water balance in Bolivian Andes, J. Disaster Res., 11, 1040–1051, https://doi.org/10.20965/jdr.2016.p1040, 2016.
Ministerio de Medio Ambiente (MMAyA), Agencia Catalana de Cooperación al Desarrollo (ACCD)/Agencia Catalana del Agua (ACA): Enfoque estratégico para la implementación de la Cuenca pedagógica del río Katari. La Paz, Bolivia [Ministry of the Environment (MMAyA), Catalan Agency for Development Cooperation (ACCD)/Catalan Water Agency (ACA) Strategic approach for the implementation of the Katari River Educational Basin, La Paz, Bolivia], https://repo.gestionintegralagua.bolivia.bo/items/show/154 (last access: 10 August 2022), 2010.
Ministerio de Medio Ambiente (MMAyA): Informe de Auditoría sobre el Desempeño Ambiental respecto de la Contaminación Hídrica en la Cuenca del Río Katari y la Bahía de Cohana K2/AP05/J13 J13 [Audit report on environmental performance regarding water pollution in the Katari River Basin and Cohana Bay K2/AP05/J13], https://datos.siarh.gob.bo/biblioteca (last access: 10 August 2022), 2014a.
Ministerio de Medio Ambiente (MMAyA): Plan Maestro Metropolitano de Agua Potable y Saneamiento La Paz-El Alto Bolivia. Ministerio de Medio Ambiente y Agua, Viceministerio de Recursos Hídricos y Riego, La Paz, Bolivia [Metropolitan Master Plan for Drinking Water and Sanitation La Paz-El Alto, Bolivia, Ministry of Environment and Water, Vice Ministry of Water Resources and Irrigation, La Paz, Bolivia], 106–172, https://datos.siarh.gob.bo/biblioteca (last access: 10 August 2022), 2014b.
Ministerio de Medio Ambiente (MMAyA): Plan Nacional de Cuencas. Ministerio de Medio Ambiente y Agua Viceministerio de Recursos Hídricos y Riego (VRHR), La Paz, Bolivia [National Basin Plan. Ministry of Environment and Water Vice Ministry of Water Resources and Irrigation (VRHR), La Paz, Bolivia], https://cdrnbolivia.org/recursos-hidricos.htm (last access: 12 August 2022), 2014c.
Ministerio de Medio Ambiente (MMAyA): Resumen Balance Hídrico Superficial Plan Director Cuenca Katari, Ministerio de Medio Ambiente y Agua Viceministerio de Recursos Hídricos y Riego (VRHR), La Paz, Bolivia [Summary of Surface Water Balance Katari Basin Master Plan, Ministry of Environment and Water Vice Ministry of Water Resources and Irrigation (VRHR), La Paz, Bolivia], 2015a.
Ministerio de Medio Ambiente (MMAyA): Sistema de monitoreo de la calidad hídrica en la Cuenca del río Katari. Ministerio de Medio Ambiente y Agua Viceministerio de Recursos Hídricos y Riego (VRHR), La Paz, Bolivia [Water quality monitoring system in the Katari River Basin, Ministry of Environment and Water Vice Ministry of Water Resources and Irrigation (VRHR), La Paz, Bolivia], 2015b.
Ministerio de Medio Ambiente (MMAyA): Plan de Manejo Preliminar de los Acuíferos de Purapurani y Viacha. Ministerio de Medio Ambiente y Agua Viceministerio de Recursos Hídricos y Riego (VRHR), La Paz, Bolivia (pp. 184) [Preliminary Management Plan for the Purapurani and Viacha Aquifers. Ministry of Environment and Water Vice Ministry of Water Resources and Irrigation, La Paz, Bolivia], https://datos.siarh.gob.bo/biblioteca (last access: 10 August 2022), 2016.
Ministerio de Medio Ambiente (MMAyA): Atlas uso de la tierra cuenca Katari y lago menor del Titicaca. Ministerio de Medio Ambiente y Agua – Ministerio de Medio Ambiente y Agua, La Paz, Bolivia, [Atlas land use Katari basin and minor Lake Titicaca], https://datos.siarh.gob.bo/biblioteca (last access: 10 August 2022), 2018.
Naciones Unidas para el Medio Ambiente (UNEP): Diagnóstico Ambiental del Sistema Titicaca-Desaguadero-Poopo-Salar de Coipas a (Sistema TDPS), División de Aguas Continentales, Programa de la UNEP. Bolivia-Perú, [Environmental Diagnosis of the Titicaca-Desaguadero-Poopo-Salar de Coipas System (TDPS System), Inland Water Division, United Nations Environment Program (UNEP), Bolivia-Peru], 1996.
Observatorio Regional de Planificación para el Desarrollo (CEPAL): Informe Nacional sobre la gestión del agua en Bolivia. La Paz, Bolivia [Observatory for Development Planning (CEPAL): National Report on water management in Bolivia, Regional, La Paz, Bolivia], 2000.
Paz, O.: Valoración de Metales Pesados en la Cuenca del río Katari y su impacto en la calidad de vida el área de influencia, 281–293, ISBN 4-1-1404-13, 2009.
Quino, I., Ormachea, M., Ramos, O. E., Bhattacharya, P., Quispe, R., Quintanilla, J., and Sracek, O.: Hydrochemical assessment with respect to arsenic and other trace elements in the Lower Katari Basin, Bolivian Altiplano, Groundwater for Sustainable Development, 8, 281–293, https://doi.org/10.1016/j.gsd.2018.11.013, 2019.
Salvarredy-Aranguren, M. M., Probst, A., Roulet, M., and Isaure, M.-P.: Contamination of surface waters by mining wastes in the Milluni Valley (Cordillera Real, Bolivia): mineralogical and hydrological influences, Appl. Geochem., 23, 1299–1324, https://doi.org/10.1016/j.apgeochem.2007.11.019, 2008.
Schroeder, P. R., Dozier, T. S., Zappi, P. A., Mcenroe, B. M., Sjostrom, J. W., and Peyton, R. L.: The hydrologic evaluation of landfill performance (Help) model, https://www.nrc.gov/docs/ML1015/ML101590183.pdf (last access: 12 August 2022), 1994.
Serrano-Juan, A., Criollo, R., Vázquez-Suñé, E., Alcaraz, M., Ayora, C., Velasco, V., and Scheiber, L.: Customization, extension and reuse of outdated hydrogeological software, Geol. Acta, 18, 1–11, https://doi.org/10.1344/GeologicaActa2020.18.9, 2020.
Tubau, I., Vázquez-Suñé, E., Carrera, J., Valhondo, C., and Criollo, R.: Quantification of groundwater recharge in urban environments, Sci. Total Environ., 592, 391–402, https://doi.org/10.1016/j.scitotenv.2017.03.118, 2017.
Valdivielso, S., Hassanzadeh, A., and Vázquez-Suñé, E., Custodio, E., Criollo, R.: Spatial distribution of meteorological factors controlling stable isotopes in precipitation in Northern Chile, J. Hydrol., 605, 127380, https://doi.org/10.1016/j.jhydrol.2021.127380, 2022.
Velasco, V., Vázquez-Suñé, E., Criollo, R., Alcaraz, M., Serrano-Juan, A., and García-Gil, A.: GIS-based hydrogeological database and analysis tools, INFOCOMP 2013, IARIA, ISBN 978-1-61208-310-0, 2013.
World Health Organization (WHO): Guidelines for drinking-water quality, 4th edition, incorporating the 1st addendum, ISBN 978-92-4-154995-0 https://www.who.int/publications/i/item/9789241549950 (last access: 12 August 2022), 2017.