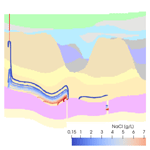
Upwelling mechanisms of deep saline waters via Quaternary erosion windows considering varying hydrogeological boundary conditions
Elena Chabab
Michael Kühn
Thomas Kempka
Intrusion of deep saline waters into freshwater aquifers does not only endanger the regional drinking water supply, but also rivers and stagnant waters and their fauna are threatened by salinisation. The upwelling of highly mineralised saline waters in large parts of the North German Basin is favoured by the presence of Elsterian glacial erosion windows in the Lower Oligocene Rupelian Clay, the most important hydraulic confining unit in this region. Lower precipitation rates and decreasing groundwater levels as a consequence of global climate change, but also anthropogenic interventions, such as increasing extraction rates or the use of the deep geologic subsurface as a reservoir, decrease the pressure potential in the freshwater column and may possibly accelerate this primarily geogenic salinisation process in the coming years. Density-driven flow and transport modelling was performed in the scope of the present study to investigate the upwelling mechanisms of deep saline waters across Quaternary window sediments in the Rupelian. Simulation results show that the interactions between the groundwater recharge rate and anthropogenic interventions such as extraction rates of drinking water wells or the utilisation of the deep subsurface, have a significant influence on the groundwater pressure potential in the freshwater aquifer and associated saltwater upwelling. In all scenarios, salinisation is most severe in the sediments of the erosion windows. Hydraulically conductive faults also intensify salinisation if located nearside erosion windows or induce a more distributed or localised salinisation in aquifers with drinking water relevance in areas that do not intersect with erosion windows. A decline in groundwater recharge thereby significantly favours upward saltwater migration. The simulation scenarios further show that a decrease in groundwater recharge also results in freshwater salinisation occurring up to 10 years earlier, which underlines the need for waterworks to initiate effective countermeasures quickly and in time.
- Article
(4404 KB) - Full-text XML
- BibTeX
- EndNote
The occurrence of geogenic saline water in the groundwater column used for freshwater production has been a known problem in many areas of the North German Basin (NGB) for many decades. Highly mineralised water with concentrations of up to 250 g L−1 of total dissolved solids (TDS) originates from deeper aquifers, where it is formed by the dilution of Upper Permian (Zechstein) salt diapirs (Hannemann and Schirrmeister, 1998; Grube et al., 2000). Saltwater upwelling is likely favoured by the partial absence of the Lower Oligocene Rupelian Clay, the most important confining unit in the region, separating the deep saline waters from the overlying Tertiary and Quaternary freshwater aquifers used for regional drinking water supply. It is assumed that the Rupelian Clay, which reaches a thickness between 80–100 m, was not deposited in places or was subject to partly deep reaching erosion as a result of multiphase inland glaciations, which significantly shaped the landscape of the NGB during the Pleistocene. As part of the NGB, the German Federal State of Brandenburg also owes its superficial geological structure and geomorphology to the most recent inland glaciation phases and reveals parts with erosion windows in the Rupelian Clay, spatially related to areas with detectable salt concentrations in the freshwater column (Stackebrandt and Manhenke, 2004; Limberg et al., 2016; Brose and Hermsdorf, 2017). In any case, the problem of salinisation is gaining in importance under the aspect of climate change and the fact that the Berlin-Brandenburg region is already facing water scarcity. The adverse effects of intensifying climatic conditions, including longer periods of droughts, decreasing precipitation and groundwater recharge, are causing a decrease in the pressure potential in the freshwater column, potentially accelerating this primarily geogenic salinisation processes in the coming years. The Potsdam Institute for Climate Impact Research (PIK) has forecasted a significant decline in groundwater recharge from 82 mm yr−1 for the period 1991–2015 to at least 50 mm yr−1 or by ∼ 40 % for the period 2040–2050 (Gerstengarbe et al., 2003). Also, anthropogenic interventions such as increasing extraction rates or the use of the deep geologic subsurface pose additional risks. The utilisation of the deep subsurface as a reservoir or for gas storage is already practised worldwide on industrial-scale and is once again increasingly coming into focus as a measure to achieve climate and energy policy objectives (IPCC, 2005; UNECE, 2021; IPCC, 2022). The storage potential of the geologic layer sequence of Brandenburg arises from the varied geological development of the NGB and its various sedimentation cycles, and is particularly high in the sandstone sequence of the Middle Buntsandstein and Keuper (Höding et al., 2009; Martens et al., 2012; Kempka et al., 2015). However, the injection of liquids or gases into the water-saturated subsurface – e.g. a saline aquifer – for long-term storage, causes elevated pressures and resident saline formation fluids might be displaced upwards (Tillner et al., 2016; Wetzel and Kühn, 2016; Tillner et al., 2013).
The present study uses density-driven flow and transport modelling to investigate upwelling mechanisms of deep saline waters across Quaternary window sediments in the Rupelian Clay in Brandenburg under the impact of groundwater extraction, a decline in groundwater recharge, elevated pressures as a result of subsurface utilisation and the presence of hydraulically conductive faults. Along a 55 km long transect extending from NE to SW, a 2D geological model was developed and extracted from a previously implemented static 3D hydrogeological model, covering the central to south-eastern part of Brandenburg (Fig. 1). The region is characterised by four major regional fault zones consisting of individual faults which result from complex Late Mesozoic and neotectonic (re-)activation phases and the development of the Middle German Crystalline-High (Beutler and Stackebrandt, 2012; Stackebrandt and Manhenke, 2004). A 2D model was applied in the present sensitivity study to first determine the key parameters relevant to deep saline water upwelling at feasible computational demands.
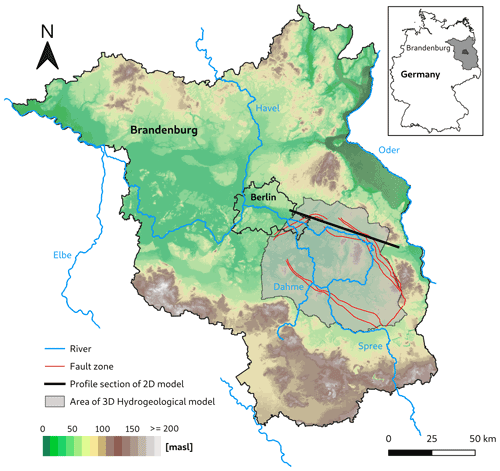
Figure 1Study area located in the south-east of the German Federal State of Brandenburg. The 2D geological model is extracted from a 3D hydrogeological model along a 55 km long transect (black line). Federal state borders, fault zones and rivers derived from Stackebrandt and Manhenke (2004). Digital Terrain Model DGM200: © GeoBasis-DE/BKG 2015.
The hydrogeological model extends over a length of 55 km and to a depth of 320 m. It contains the Rupelian sequence with saline basal sands as the model basis and overlying Rupelian Clay as the main confining unit to the freshwater column. The Tertiary pore aquifers and Quaternary unconsolidated rock series above comprise the most important freshwater aquifer complexes for drinking water supply in the region (Fig. 2). Hydrostratigraphy and lithology of the model are based on a static 3D hydrogeological model that was implemented using geologic and hydrogeologic maps as well as hydrogeologic sections and, in addition, stratigraphic logs from borehole profiles (LBGR, 1994–2013; LBGR, 1982–1988). The final 2D model grid contains 87 200 hexahedron elements in total (nx = 545, ny = 1, nz = 160) with a resolution of dx = 100, dy = 1, dz = 2 m. Three erosion windows in the Lower Oligocene Rupelian Clay filled with Quaternary sediments and an average cross-sectional width between 1.7 and 2.5 km are present along the chosen profile section.
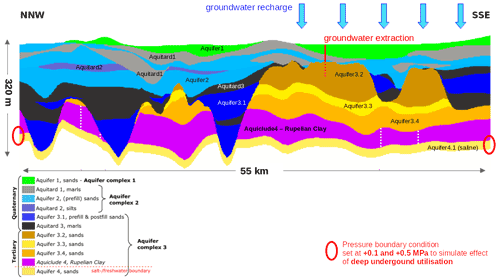
Figure 22D model applied in all simulations. Model base is the saline Rupelian basal sand below the main aquiclude in the region, the Lower Oligocene Rupelian Clay. The freshwater column composes a strata of sands (and gravels) with intercalated marly tills and clay beds of Tertiary and Quaternary age. The aquifer complexes 2 and 3 hold important freshwater reservoirs used for regional drinking water supply. Fault locations are indicated by light yellow dashed lines.
For the parametrisation of the hydrogeological model, strata-uniform permeability coefficients according to Zippel (2005) were assumed (based on Voigt, 1987; GCI, 1998; FUGRO, 1998). Values for the effective porosity of the hydrogeological units are based on petrophysical properties according to Matthess and Ubell (1983). In all numerical simulations, a salt concentration of 10 g L−1 is assumed for the saline basal sands at the base of the Rupelian Clay (Aquifer 4.1; Grube et al., 2002; Kempka et al., 2015). Table 1 lists all scenarios considered in this study. In four scenarios, the effect of elevated pressures in the saline basal sands of 0.1 and 0.5 MPa resulting from hypothetical subsurface utilisation was investigated. This is implemented in the models via a pressure boundary condition in the respective aquifer (Aquifer 4.1) and applied to both boundaries to assess the effect on saline water migration across a nearside and more distant erosion window. The influence of groundwater extraction is examined in two scenarios. The local waterworks Fürstenwalde extracts in the region from the Tertiary Aquifer 3.2 via five wells. This well gallery is represented by a single well in the model and the mean daily pumping rate is adjusted to the model dimensions. In order to determine the impact of a decline in groundwater recharge on freshwater salinisation, all scenarios were calculated with a rate of 82 mm yr−1 and a 40 % reduction to 50 mm yr−1, adjusted to the model dimensions. Precipitation reaches its highest values in the SE on the Lieberos Plateau, which is why groundwater recharge is limited to the central to the SE part of the model area, also due to the connectivity to the underlying aquifers in the 2D section. All simulations were carried out with and without hydraulically conductive faults intersecting the Rupelian sequence, and parametrised identical to the saline basal sands (Aquifer 4.1) in a conservative approach, however, without an initial salt concentration. The simulations were undertaken using the TRANSPORT Simulation Environment (TRANSPORTSE) which is a density-driven flow and transport simulator (Kempka, 2020). A simulation time of 100 years was applied in all scenarios.
Table 1Scenarios 1–4 investigate the impact of an increased pressure gradient due to a hypothetical subsurface utilisation, Scenarios 5–6 consider the impacts of groundwater extraction on upper aquifer salinisation. In every scenario, a decline in groundwater recharge by 40 % and the presence of hydraulically conductive faults is taken into account.
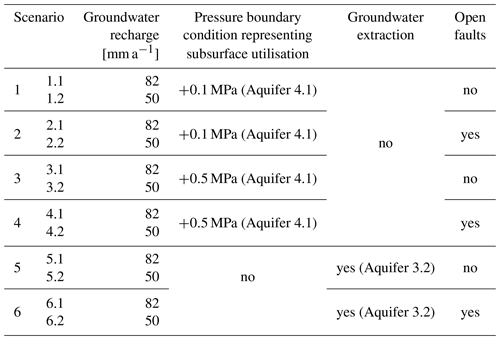
The scenarios considering geologic subsurface utilisation show that at smaller distances from an erosion window to a local pressure pulse, the saltwater rises considerably higher in the erosion windows than at greater distances. In this context, the saltwater rise also depends on how strongly the pressure has increased and how long this increase persists. In case of a pressure increase of 0.5 MPa at a distance of 1 km to an erosion window, the salt-/freshwater boundary is shifted upwards via the erosion window into the freshwater column by 230 m. In a more distant erosion window, 24 km from the largest pressure increase, the salt-/freshwater boundary rises by 73 m (Scenario 3.2; Fig. 3). The extent of the saltwater-influenced area herein and in the following refers to a minimum salt concentration of 0.15 g L−1. This orientation value can be used as an indicator for geogenic salinisation of groundwater in Brandenburg (Brose and Hermsdorf, 2017). In total, a salt mass of 404 Mg m−1 model thickness perpendicular to the profile section is displaced into freshwater Aquifer 2 after 100 years of geologic subsurface utilisation and under the assumption of a groundwater recharge of 82 mm yr−1 (Scenario 3.2; Fig. 4). With a decline in groundwater recharge by about 40 % to 50 mm yr−1, an additional 14 Mg m−1 NaCl reaches Aquifer 2 (Scenario 3.1; Fig. 4). If the erosion window is located in the area of a hydraulically conductive fault, the salinisation is further intensified in the area. In total, 482 Mg m−1 NaCl (Scenario 4.2) and 491 Mg m−1 NaCl (Scenario 4.1) reach Aquifer 2 after 100 years, assuming a groundwater recharge of 82 and 50 mm yr−1, respectively (Figs. 3 and 4). Hydraulically conductive faults can also induce localised salinisation in aquifers with drinking water relevance and areas that do not intersect with erosion windows. In Scenarios 4.1 and 4.2, salinisation therefore also affects Aquifers 3.4, 3.3 and 3.2, which are located above two geologic faults. The relative salt mass change in these aquifers estimates 515 Mg m−1 (Aquifer 3.4), 34 Mg m−1 (Aquifer 3.3) and 8 Mg m−1 (Aquifer 3.2) after 100 years and a groundwater recharge of 82 mm yr−1 (Scenario 4.2, Fig. 4). In case of a decline in groundwater recharge to 50 mm yr−1, the relative salt mass change increases by 7 % to 551 Mg m−1 (Aquifer 3.4), 143 % to 80 Mg m−1 (Aquifer 3.3) and 330 % to 27 Mg m−1 (Aquifer 3.2) after 100 years (Scenario 4.1, Fig. 4). Even with a pressure increase of only 0.1 MPa near the erosion windows or faults, simulations show that the saltwater can rise by 165 m, however, limited to the Quaternary fillings of the erosion window (Aquifer 3.1, Aquitard 3). For erosion windows further away from the pressure boundary, salinisation is concentrated mainly in the Quaternary window sediments (Aquifer 3.1 and Aquitard 3; Fig. 4), as it is also the case for a pressure increase of 0.5 MPa.
The scenarios with groundwater extraction from Aquifer 3.2 demonstrate that due to water production, the salt-/freshwater boundary is shifted significantly upwards, however, salinisation is mainly limited to the erosion windows. Thus, the highest relative salt mass changes can be observed in Aquifer 3.1 in all scenarios (Figs. 5 and 6). In case of closed faults, 504 Mg m−1 NaCl are transported into Aquifer 3.1 after 100 years, assuming a groundwater recharge of 82 mm yr−1 (Scenario 5.1). With a reduced groundwater recharge of 50 mm yr−1, the relative salt mass change is 12 % higher, with a total of 568 Mg m−1 NaCl reaching the Quaternary window sediments of Aquifer 3.1 (Scenario 6.1; Fig. 6). The smaller the distance between erosion window and production well, the further the salt-/freshwater boundary is shifted upwards via the erosion window. In the erosion window closest to production saltwater has risen by 66 m after 100 years of production and by 118 m in the erosion window furthest away (e.g. Scenario 5.1; Fig. 6). A decrease in groundwater recharge (Scenario 6.1) results in the Tertiary freshwater Aquifer 3.4 above the Rupelian Clay being affected by salinisation a few years earlier than in Scenario 5.1 due to saltwater migration across the central erosion window. After 100 years of production, 0.9 Mg m−1 NaCl were transported into Aquifer 3.4 at a groundwater recharge of 82 mm yr−1. With 5 Mg m−1, the salt mass transported into Aquifer 3.4 is five times higher for the same period, given the lower groundwater recharge. However, neither other shallower Tertiary or Quaternary aquifers nor the near-field of the production well are affected by salinisation in case of closed faults. In case of open faults, again the largest relative salt mass changes can be observed in the erosion windows itself (Aquifer 3.1) with 464 Mg m−1 NaCl in total (Scenario 6.2; Fig. 6). Compared to closed faults, however, the amount of transported salt via the window sediments is lower overall as the faults also serve as migration paths, leading to a more distributed salinisation pattern in the freshwater column. A decline in groundwater recharge results in an increase of transported salt mass into the Quaternary window sediments by 22 % to 568 Mg m−1 (Scenario 5.2; Fig. 6). Aquifers relevant for drinking water supply in the SE are much more vulnerable to salinisation, because of the location of the faults. The saltwater reaches Aquifer 3.4 after 4 years and the more shallower Aquifer 3.2 and production well after 80 years. If groundwater recharge decreases, Aquifer 3.4 is affected by salinisation already 1.5 years earlier and the production after 70 years. The total amount of salt being displaced into Aquifer 3.4 after 100 years amounts to 116 Mg m−1 (Scenario 6.2) and is again 53 % higher with 177 Mg m−1 (Scenario 5.2) for the lower groundwater recharge. In all scenarios with open faults, Quaternary Aquifer 2 remains unaffected by salinisation.
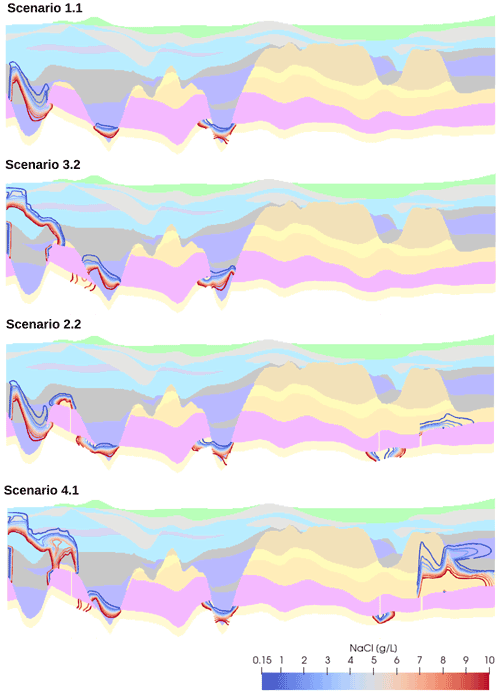
Figure 3Contour lines showing the maximum extent of salinisation after 100 years for the geologic subsurface utilisation Scenarios 1.1, 3.2, 2.2 and 4.1. The minimum salt concentration plotted is 0.15 g L−1 as an indicator for geogenic salinisation in Brandenburg (Brose and Hermsdorf, 2017). For the legend of the hydrostratigraphy, please refer to Fig. 2.
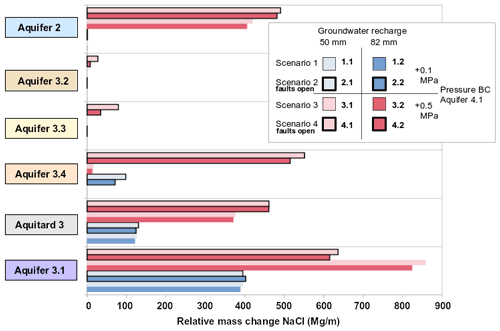
Figure 4Relative salt mass change in the aquifers above the Rupelian for the geologic subsurface utilisation scenarios. Aquifers or aquitards with negligible salt mass changes are not shown.
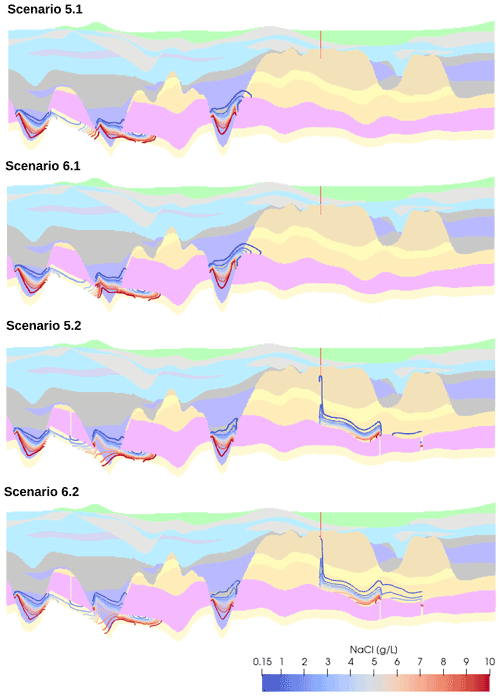
Figure 5Contour lines showing the maximum extent of salinisation after 100 years for the groundwater extraction scenarios 5.1, 5.2, 6.1 and 6.2. The minimum salt concentration plotted is 0.15 g L−1 as an indicator for geogenic salinisation in Brandenburg (Brose and Hermsdorf, 2017). For the legend of the hydrostratigraphy, please refer to Fig. 2.
Groundwater salinisation is a major challenge for sustainable water management in many regions, including the German Federal State of Brandenburg. The present study uses density-driven flow and transport modelling to investigate upwelling mechanisms of deep saline waters across Quaternary window sediments in the Lower Oligocene Rupelian Clay in Brandenburg. For this purpose, a 2D hydrogeological model was constructed along a 55 km long transect based on local geology and hydrostratigraphy to examine the relevance of groundwater extraction, decline in groundwater recharge, increased pressure gradients due to geologic subsurface utilisation and the presence of hydraulically conductive faults on the occurrence of saltwater in the freshwater column in various modelling scenarios.
The scenarios with geologic subsurface utilisation show that the degree of upper aquifer salinisation depends primarily on the proximity of the pressure increase to the erosion window in the Rupelian Clay. If the pressure increase resulting from subsurface utilisation persists for many years, significant amounts of salt can reach the freshwater column via the erosion windows. In this context, the saltwater rise accordingly also depends on how strongly the pressure is increased. However, even a pressure increase of 0.1 MPa near erosion windows or faults can significantly shift the salt-/freshwater boundary upwards by several tens to hundreds of metres. However, salinisation is concentrated mainly in the sediments of the window itself in more distant erosion windows. If the erosion window is located in the area of a hydraulically conductive fault, salinisation may be further intensified in that area. Hydraulically conductive faults can also induce a more distributed or localised salinisation in aquifers with drinking water relevance in areas that do not intersect with erosion windows, which makes it important to consider the system as a whole with all its hydrogeological and structural geological conditions. Groundwater extraction also causes saltwater to enter the shallower aquifers via erosion windows and faults, but more severe salinisation occurs only after a longer time period.
Groundwater recharge has proven to be a sensitive parameter and its decline significantly promotes upward saltwater migration in all investigated scenarios. In the deeper aquifers, a decreasing groundwater recharge has only a minor effect on salinisation. However, the shallower the groundwater units, the greater the noticeable changes, with relative salt mass changes in the freshwater column that are up to five times higher compared to the scenarios with 40 % higher groundwater recharge. A decline in groundwater recharge also has the effect that salinisation of freshwaters occurs much sooner, so that local waterworks have to initiate effective countermeasures at an earlier stage. Saltwater upconing, i.e. the vertical movement of saline water from deep saline aquifers into the freshwater column and the formation of a saline water plume in the form of a cone below production wells as a result of groundwater extraction, is a well-known problem in many coastal and inland aquifers and has been analysed by several authors using analytical solutions or numerical simulations (Chandler and McWhorter, 1975; Reilly and Goodman, 1987; Zhou et al., 2005; Cai et al., 2014; Pauw et al., 2015) as well as at the laboratory scale (Johannsen et al., 2002; Jakokovic et al., 2011). The investigations point to the necessity of appropriate water production management strategies and optimum aquifer penetration to avoid critical saltwater rise. In their investigations on saltwater upconing in the German Federal State of Brandenburg, Voss and Koch (2001) show by means of density-driven flow and transport models that upconing also occurs below the discharge areas of local rivers. However, effects of water production were neglected in the modelling study and the authors state that a fully 3D density-dependent groundwater flow and transport model is required to account for the spatial variation of flow patterns and hydraulically active fluid flow conduits.
The present study highlights the impact of different (hydrogeological) boundary conditions on saltwater migration across Quaternary erosion windows in the Lower Oligocene Rupelian Clay in Brandenburg and reveals significant potential consequences for the water supply and quality of drinking water aquifers, especially with regard to lowering groundwater tables as a result of predicted declining recharge rates. The results can thus provide a scientific contribution to the identification of adaptation and countermeasures to support the development and implementation of a sustainable water management in Brandenburg. For future investigations, it is planned to extend the presented 2D simulation study to the third dimension to account for the aforementioned 3D flow effects on the timely and spatial distribution of upper aquifer salinisation due to spatial variations in groundwater recharge and the thickness and expansion of the respective layer sequences and erosion windows, as well as changing fault strikes. In addition, multiple groundwater production wells will be included in the simulations to represent entire well galleries, with spatially and temporally varying or intermitted pumping rates, as well as receiving water bodies such as rivers and lakes. Further, the equation of state will be extended to account for the effects of the conditions on fluid density and viscosity (Kühn et al., 2022; Otto et al., 2022).
Please contact the author for information on code availability.
The data will be only made available on specific user requests.
Conceptualisation: EC and TK; Software development: TK, Simulations: EC; Result and data analysis: EC and TK; Graphical illustrations: EC; Writing and internal review: EC, TK and MK.
The contact author has declared that none of the authors has any competing interests.
Publisher’s note: Copernicus Publications remains neutral with regard to jurisdictional claims in published maps and institutional affiliations.
This article is part of the special issue “European Geosciences Union General Assembly 2022, EGU Division Energy, Resources & Environment (ERE)”. It is a result of the EGU General Assembly 2022, Vienna, Austria, 23–27 May 2022.
We thank two anonymous reviewers for their valuable comments that helped to improve the quality of the manuscript.
This publication has been supported by the funding programme “Open Access Publikationskosten” Deutsche Forschungsgemeinschaft (DFG, German Research Foundation (project number 491075472)). The article processing charges for this open-access publication were covered by the Helmholtz Centre Potsdam – GFZ German Research Centre for Geosciences.
This paper was edited by Christopher Juhlin and reviewed by two anonymous referees.
Beutler, G. and Stackebrandt, W.: The tectonic pattern of the sedimentary cover of Brandenburg – suggestion for a uniform nomenclature, Brandenburg. Geowiss. Beitr., 19, 93–109, 2012 (in German).
Brose, D. and Hermsdorf, A.: Geogenic salinization in aquifers of Brandenburg, Brandenburg. Geowiss. Beitr., 24, 7–16, 2017 (in German).
Cai, J., Taute, T., and Schneider, M.: Saltwater Upconing Below a Pumping Well in an Inland Aquifer: a Theoretical Modeling Study on Testing Different Scenarios of Deep Saline-Groundwater Pathways, Water Air Soil Pollut., 225, 2203, https://doi.org/10.1007/s11270-014-2203-7, 2014.
Chandler, R. and McWhorter, D.: Upconing of the salt-water-fresh-water interface beneath a pumping well, Ground Water, 13, 354–359, https://10.1111/j.1745-6584.1975.tb03599.x, 1975.
FUGRO GmbH Berlin: Grundlagenermittlung zur Stofftransportmodellierung Schwarze Pumpe – Tabelle 4, Berlin, 1998 (unpublished, in German).
GCI (Grundwasser Consulting Ingenieurgesellschaft mbH): Hydrogeologisches Strukturmodell Wasserwerke Johannisthal/Altglienicke, Gutachten für BWB; Berlin, 1998 (unpublished, in German).
Gerstengarbe, F., Badeck, F.-W., Hattermann, F., Krysanova, V., Lahmer, W. Lasch, P., Stock, M., Suckow, F., Wechsung, F., and Werner, P.: Studie zur klimatischen Entwicklung im Land Brandenburg bis 2055 und deren Auswirkungen auf den Wasserhaushalt, die Forst- und Landwirtschaft sowie die Ableitung erster Perspektive, PIK Report No. 83, p. 79, 2003 (in German).
Grube, A., Wichmann, K., Hahn, J., and Nachtigall K.H.: Geogene Grundwasserversalzung in den Porengrundwassserleitern Norddeutschlands und ihre Bedeutung für die Wasserwirtschaft, Technologiezentrum Wasser Karlsruhe (TZW), Karlsruhe, p. 20, 2000 (in German).
Grube, A., Hermsdorf, A., Lang, M., Rechlin, B., and Steffens, A.: Numeric and Hydrochemical Modelling of Saltwater Intrusion into a Pleistocene Aquifer – Case Study Großbeuthen (Brandenburg), 17th Salt Water Intrusion Meeting, Delft, the Netherlands, 6–10 May 2002, p. 12, 2002.
Hannemann, M. and Schirrmeister W.: Paläahydrogeologische Grundlagen der Entwicklung der Süß-/Salzwassergrenze und der Salzwasseraustritte in Brandenburg, Brandenburg. Geowiss. Beitr., 5, 61–72, 1998 (in German).
Höding, T., Göthel, M., and Stackebrandt, W.: Geological opportunities for underground storage of CO2 in Brandenburg (Germany), Brandenburg. Geowiss. Beitr., 16, 1–18, 2009 (in German).
IPCC: Special Report on Carbon Dioxide Capture and Storage, Prepared by Working Group III of the Intergovernmental Panel on Climate Change, edited by: Metz, B., Davidson, O., de Coninck, H. C., Loos, M., and Meyer, L. A., Cambridge University Press, Cambridge, United Kingdom and New York, NY, USA, 442 pp., ISBN 978-0-521-86643-9, 2005.
IPCC: Climate Change 2022: Mitigation of Climate Change, Working Group III contribution to the Sixth Assessment Report of the Intergovernmental Panel on Climate Change, p. 2913, https://report.ipcc.ch/ar6/wg3/IPCC_AR6_WGIII_Full_Report.pdf, last access: 19 July 2022.
Jakovovic, D., Werner, A. D., and Simmons, C. T.: Numerical modelling of saltwater up-coning: comparison with experimental laboratory observations, J. Hydrol., 402, 261–273, https://doi.org/10.1016/j.jhydrol.2011.03.021, 2011.
Johannsen, K., Kinzelbach, W., Oswald, S., and Wittum, G.: The saltpool benchmark problem – numerical simulation of saltwater upconing in a porous medium, Adv. Water Resour., 25, 335–348, https://doi.org/10.1016/S0309-1708(01)00059-8, 2002.
Kempka, T.: Verification of a Python-based TRANsport Simulation Environment for density-driven fluid flow and coupled transport of heat and chemical species, Adv. Geosci., 54, 67–177, https://doi.org/10.5194/adgeo-54-67-2020, 2020.
Kempka, T., Herd, R., Huenges, E., Endler, R., Jahnke, C., Janetz, S., Jolie, E., Kühn, M., Magri, F., Meinert, P., Moeck, I., Möller, M., Muñoz, G., Ritter, O., Schafrik, W., Schmidt-Hattenberger, C., Tillner, E., Voigt, H.-J., and Zimmermann, G.: Joint Research Project Brine: Carbon Dioxide Storage in Eastern Brandenburg: Implications for Synergetic Geothermal Heat Recovery and Conceptualization of an Early Warning System Against Freshwater Salinization, in: Geological Storage of CO2 – Long Term Security Aspects, edited by: Liebscher, A. and Münch, U., GEOTECHNOLOGIEN Science Report No. 22, Advanced Technologies in Earth Sciences, Springer International Publishing, 139–166, https://doi.org/10.1007/978-3-319-13930-2_9, 2015.
Kühn, M., Präg, M., Becker, I., Hilgers, C., Grafe, A., and Kempka, T.: Geographic Information System (GIS) as a basis for the next generation of hydrogeological models to manage the geothermal area Waiwera (New Zealand), Adv. Geosci., 58, 31–39, https://doi.org/10.5194/adgeo-58-31-2022, 2022.
LGBR (Landesamt für Bergbau, Geologie und Rohstoffe Brandenburg): HYK50 – Hydrogeologische Schnitttafeln, Hydrogeologisches Kartenwerk Brandenburg 1:50 000, 1994–2013 (in German).
LGBR (Landesamt für Bergbau, Geologie und Rohstoffe Brandenburg): GK200 – Geologische Schnitttafeln, Geologische Karte der ehemaligen DDR, 1:200 000, 1972–1990 (in German).
Limberg, A., Jonas, O., and Kolberg, A.: Detektion möglicher Fehlstellen im Rupelton durch Messung der spezifischen elektrischen Leitfähigkeit in tiefen Grundwassermessstellen im Land Berlin, Brandenburg. Geowiss. Beitr., 23, 11–15, 2016 (in German).
Martens, S., Kempka, T., Liebscher, A., Lüth, S., Möller, F., Myrttinen, A., Norden, B., Schmidt-Hattenberger, C., Zimmer, M., Kühn, M., and the Ketzin Group: Europe's longest-operating onshore CO2 storage site at Ketzin, Germany: A progress report after three years of injection, Environ. Earth Sci., 67, 323–334, https://doi.org/10.1007/s12665-012-1672-5, 2012.
Matthess, G. and Ubell, K.: Allgemeine Hydrogeologie, Grundwasserhaushalt, in: Lehrbuch der Hydrogeologie, Herausgeber*innen: Matthess, G., Stuttgart, Gebrüder Borntraeger, ISBN 978-3-443-01005-8, 1983 (in German).
Otto, C., Steding, S., Morgan, M., Gorka, T., Hámor-Vidó, M., Basa, W., Kapusta, K., Kalmár, I., and Kempka, T.: Probability of contaminant migration from abandoned in-situ coal conversion reactors, Adv. Geosci., 58, in press, 2022.
Pauw, P. S., van der Zee, S. E., Leijnse, A., and Oude Essink, G. H.: Saltwater Upconing Due to Cyclic Pumping by Horizontal Wells in Freshwater Lenses, Groundwater, 54, 521–531, https://doi.org/10.1111/gwat.12382, 2015.
Reilly, T. E. and Goodman, A. S.: Analysis of saltwater upconing beneath a pumping well, J. Hydrol., 89, 169–204, https://doi.org/10.1016/0022-1694(87)90179-X, 1987.
Stackebrandt, W. and Manhenke, V. (Eds.): Atlas zur Geologie von Brandenburg, Landesamt für Bergbau, Geologie und Rohstoffe (LBGR), 2004 (in German).
Tillner, E., Kempka, T., Nakaten, B., and Kühn, M.: Brine migration through fault zones: 3D numerical simulations for a prospective CO2 storage site in Northeast Germany, Int. J. Greenh. Gas Control., 19, 689–703, https://doi.org/10.1016/j.ijggc.2013.03.012, 2013.
Tillner, E., Wetzel, M., Kempka, T., and Kühn, M.: Fault damage zone volume and initial salinity distribution determine intensity of shallow aquifer salinisation in subsurface storage, Hydrol. Earth Syst. Sci., 20, 1049–11067, https://doi.org/10.5194/hess-20-1049-2016, 2016.
UNECE: Technology Brief – Carbon Capture, Use and Storage (CCUS), United Nations publication issued by the United Nations Economic Commission for Europe, United Nations publications, p. 32, https://unece.org/sites/default/files/2021-03/CCUS_brochure_EN_final.pdf (last access: 21 July 2022), 2021.
Voigt, H. J.: ZGI: Hydrogeologisches Kartenwerk der DDR 1:50000 (HK50) – Nutzerrichtlinie – Hrsg.: VEB Hydrogeologie, Nordhausen, 1987 (unpublished, in German).
Voss, A. and Koch, M.: Numerical Simulations of Topography-Induced Saltwater Upconing in the State of Brandenburg, Germany, Phys. Chem. Earth, 26, 353–359, https://doi.org/10.1016/S1464-1909(01)00018-1, 2001.
Wetzel, M. and Kühn, M.: Salinization of Freshwater Aquifers Due to Subsurface Fluid Injection Quantified by Species Transport Simulations, Energy Procedia, 97, 411–1418, https://doi.org/10.1016/j.egypro.2016.10.036, 2016.
Zhou, Q. L., Bear, J., and Bensabat, J.: Saltwater upconing and decay beneath a well pumping above an interface zone, Transp. Porous Media, 61, 337–363, https://doi.org/10.1007/s11242-005-0261-4, 2005.
Zippel, M.: Modellgestützte Bilanzierung der unterirdischen Wasserressourcen Berlins, die Grundwassersituation im weiteren Einzugsgebiet des Müggelsees, Dissertation. Freie Universität Berlin, Berlin, https://doi.org/10.17169/refubium-12303, 2005 (in German).