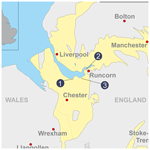
Using core and outcrop analogues to predict flow pathways in the subsurface: examples from the Triassic sandstones of north Cheshire, UK
Joanna Thompson
Daniel Parkes
Edward Hough
Oliver Wakefield
Borehole core provides detailed vertical data which is used to interpret subsurface sand body architectures, but assumptions are made on the relationship between the lateral and vertical thickness, and the interconnectivity of units. The sedimentological complexity of the Sherwood Sandstone Group succession in this area, passing between aeolian and fluvial packages creates local- to regional-scale heterogeneities which will impact flow pathways within the rockmass. Measured thickness in boreholes might represent an architectural element's true maximum thickness or more likely, a partial thickness as a result of incision by overlying facies types or as a result of the borehole sitting towards the margins of individual elements (e.g. tapering margin of channel elements). Length and thickness data were measured from a suite of primary core data and secondary published outcrop studies in north-west England. The addition of outcrop studies in combination with the borehole data provides a dataset from which the likely lateral extent of the architectural frameworks within the Triassic sandstones can be extrapolated. The interpreted high resolution sub-seismic architecture contributes to an increased understanding of flow pathways and the effect these may have on groundwater as well as sustainable energy technologies such as low-temperature geothermal aquifers, carbon storage and energy storage.
- Article
(3766 KB) - Full-text XML
- BibTeX
- EndNote
A thorough understanding of fluid flow in bedrock geological units is relevant to aquifer and reservoir management, allowing for optimised use of increasingly stressed subsurface resources such as pore space and heat extraction. Subsurface fluid flow is relevant to the development of new, low-carbon energy technologies (e.g., geothermal systems located in sedimentary aquifers, underground gas storage and Carbon Capture and Storage; CCS). In the UK, there is the potential for the development of these geological settings where there are porous and permeable rocks that may serve as production or storage horizons located in proximity to sources of energy such as shale gas reservoirs, coal seams or renewables; operational viability is increased where these geologies are located near areas of industrial activity or areas of dense population. One such area is in north Cheshire in north-west England, where the Sherwood Sandstone Group (SSG) aquifer overlies coal measures and the Bowland shale gas prospect, with thick beds of halite used for gas storage located within 25 km. The area is also located in the “Protos” area of industry (http://thisisprotos.com/, last access: 21 August 2019), located near Liverpool, Manchester and associated conurbations. This site has been chosen as the Cheshire Energy Research Field Site (CERFS), part of a GBP 31 million initiative to develop technologies to decarbonise energy by the UK Government (Fig. 1). It is planned that the CERFS will comprise a network of deep and shallow boreholes containing state-of-the-art monitoring equipment to measure groundwater (levels, flow, temperature and chemistry), ground motion, and seismicity. The proposed boreholes will range in drilled depth from 25 m to 1200 m (below surface), producing a dense dataset including down-hole geophysical and image logs and recover more than 3000 m of new drill core in the area of interest, largely from the Triassic SSG.
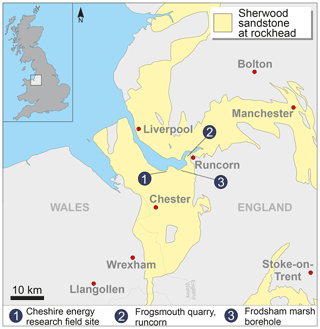
Figure 1Location map showing the generalised distribution of the SSG at rockhead. © UKRI 2019, British Geological Survey, contains Ordnance Survey data © Crown Copyright and database rights 2019 [licence number 100021290 EUL].
The SSG is a principal aquifer, a hydrocarbon reservoir offshore and onshore and a potential host for CO2 storage and aquifer geothermal schemes. The sedimentological and structural complexity of the SSG succession in this area, with interactions between aeolian and fluvial processes and development of the Cheshire Basin, creates local and regional scale heterogeneity which will impact fluid flow within the rockmass. In particular, the influence of depositional facies on the diagenetic evolution of the units is well-documented (Bouch et al., 2006), and this variation in diagenesis has a direct impact on the physical properties including porosity, permeability and fracture development (Plant et al., 1999). This is especially important when reservoir sandbodies are the subject of porosity and permeability reducing processes such as secondary mineral precipitation and deformation. As much of this heterogeneity exists on a sub-seismic scale, field-scale observations are important to understand the likely scales of variability of the sandstone in the subsurface. Aeolian units are generally thought of as having relatively high porosity and permeability; however, interdune deposits can create baffles to flow, and natural fractures within the bedrock could represent barriers to flow (e.g., deformation bands) or pathways (as in the case of some sediment-filled fractures) (Wealthall et al., 2001; Hough et al., 2006). Estimating the dimensions and distribution of the depositional elements within the sandstone will be an important input parameter to detailed flow models that give better estimates of transmissivity and fluid flow through the aquifer.
Outcrop analogue studies can be an effective method to gain information that describes the scale and relationship between different architectural elements within a stacked, multi-storey sand body (e.g., Henares et al., 2014; Marriott and Hillier, 2014). Previous studies characterising the bedrock in Runcorn, Cheshire (Lawrence et al., 2006) and Sellafield, Cumbria (e.g., Medici et al., 2016), indicate that this approach is particularly successful when applied to the SSG. Lawrence et al. (2006) proposed a classification scheme whereby the sandstone architecture of the SSG was split between “Layercake” and “Jigsaw” styles (Fig. 2) based on the lateral continuity of mudstones within the succession, and this has been used in this observational study to better understand connectivity between sandbodies in north Cheshire.
The Sherwood Sandstone Group is Early to Mid-Triassic (Induan to Anisian) in age and provides a preserved record of dryland sedimentation. During this time, rifting and extension related to the break-up of Pangea allowed thick accumulations of arenaceous sediments to develop in a series of actively subsiding linked half-grabens which fringed the local palaeo-high of the Pennines. North Cheshire lies on the Llyn-Rossendale structural high, which separates the northern part of the Cheshire Basin from the south-eastern part of the East Irish Sea Basin (Fig. 3). Locally, sediments are over 1000 m thick in some depocentres, with younger units overlapping interbasinal highs. Regional-scale depositional models suggest a southerly source in the Variscan foldbelt some few hundreds of kilometres to the south, with northwards draining rivers bifurcated by the Pennine High, diverting sediment westwards towards the Cheshire Basin, Lancashire and Cumbria, and eastwards to the Needwood Basin and East-Midlands Shelf (McKie and Williams, 2009).
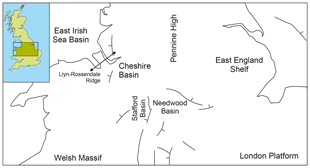
Figure 3Basin structure of the study area. © UKRI 2019, British Geological Survey, contains Ordnance Survey data © Crown Copyright and database rights 2019 [licence number 100021290 EUL].
To the east of the Pennine High, subsidence rates are thought to have been steady, allowing for the accumulation of a thick fluvial succession (Wakefield et al., 2015). This contrasts to the succession to the west of the Pennine High (including the Cheshire Basin), where the SSG comprises a series of alternating aeolian-dominated (Wilmslow Sandstone Formation and part of the Helsby Sandstone Formation) and sub-aqueous (Chester Formation and part of the Helsby Sandstone formation) units, with the SSG succession as a whole becoming increasingly aeolian upwards (Crofts et al., 2012) (Fig. 4). In many parts of central and north-west England, the fluvial units exhibit a higher degree of induration (e.g. Bridge and Hough, 2002; Howard et al., 2007; Crofts et al., 2012), and as a result may form prominent topographic highs including hills and ridges, such as the Runcorn-Frodsham-Helsby escarpment. The SSG is particularly well-exposed along these features, with numerous small quarries and natural sections allowing features of the bedrock to be measured and described. Away from these topographic highs, information describing the SSG has been obtained from borehole material.
The approach taken in this study was to establish a facies scheme from published literature and apply this to logged core of the Frodsham Marsh Borehole, located close to the proposed CERFS field research site.
3.1 Facies Scheme
Four studies have classified the depositional character of the SSG in north-west England (Thompson, 1970; Steel and Thompson, 1983; Mountney and Thompson, 2002; Bloomfield et al., 2006). These have allowed the development of a framework to identify depositional units of sandstone in core and at outcrop in this study (Table 1).
Table 2Measurements and calculated ratios from Frogsmouth Quarry panels (data collated from Mountney and Thompson, 2002).
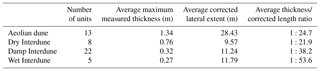
3.2 Data
The Wilmslow Sandstone Formation of the SSG is exposed in the Frogsmouth Quarry at Runcorn [BGS reference number SJ 5064 8193]. The present day quarry faces are up to 20 m high, and comprise a series of stacked aeolian dunes and interdunes in the lower part, with fluvial channel sandstones present in the upper part. Architectural panels presented by Mountney and Thompson (2002) have been analysed to give ratios of the vertical versus lateral extent of individual depositional facies of the Delamere Sandstone Member. The lateral measurement values were corrected to account for the orientation of the section to give a maximum value perpendicular to the dominant paleo-flow direction, using an average vector mean paleocurrent direction of 264∘.
The Frodsham Marsh Borehole (BGS reference number SJ47NE/14) was drilled to a depth of 117.75 m in 1983 for groundwater abstraction. The borehole intersects 75.75 m of sandstone which is also assigned to the Wilmslow Sandstone Formation of the SSG beneath 42 m of Quaternary superficial deposits. The core was logged at a nominal scale of 1:50, allowing for salient sedimentological features to be described and interpreted. The thickness of the different facies were recorded, giving the vertical extent of different depositional elements (see Facies Scheme, Table 1).
Mountney and Thompson (2002) present detailed sedimentalogical panels of the Delamere Sandstone Member of the Helsby Sandstone Formation from Frogsmouth Quarry in Runcorn, Cheshire, UK. Measurements of individual dune and interdune units as defined by the facies scheme (Table 1) were taken from these panels and corrected to give true lateral extent. Where units were complete (units were not obscured by vegetation or extended beyond the panel extent), the measurements were included in calculations to establish a ratio between thickness and lateral extent – see Table 2.
These measurements suggest a thickness progression, with units becoming thinner on average as they become wetter. A progression is also seen in the average lateral extent for each interdune type from dry to wet with the aeolian dunes extending furthest. These values are reflected in the thickness to corrected lateral extent ratios with the smallest ratio being seen in aeolian dune and dry interdune units, becoming larger in damp interdune units and larger still for wet interdune units. The larger average lateral extent of the aeolian dune units when compared with the interdune units, suggests a position towards the centre of the dunefield where the interdunes are constrained by dunes and so are unable to develop further laterally (Mountney, 2006).
An estimate of the lateral extents of the units logged from the Frodsham Marsh borehole core (Fig. 5) can be calculated (Table 3) using the ratio of the lateral extent to thickness calculated from the desk study (Table 2). Although the aeolian dune units are again the thickest units on average, the variation in thickness between the depositional elements is less pronounced. Therefore, when the ratios from the Frogsmouth Quarry measurements are applied to thicknesses taken from the Frodsham Marsh core, we see the more laterally persistent units being the damp and wet interdune units with the dry interdune units being the least laterally persistent. This suggests that the core is located in a more distal part of the dunefield with either more expansive interdunes enclosed by highly separated dunes or as isolated barchan dune types entirely surrounded by interdunes. It is also possible that more fine grained material is seen in the borehole core than in outcrop due to outcrop naturally favouring better cemented coarser material.
It is possible to measure vertical versus lateral dimensions from outcrop giving an indicative ratio of lateral extent versus thickness. This indicative ratio can be applied to vertical thicknesses recorded in borehole core logs to estimate the lateral dimensions of each unit. In this study, it has been predicted that wet and damp interdune units (i.e. potential baffles to flow) vary in lateral extent between the Frogsmouth Quarry site and the Frodsham Marsh borehole core and that this reflects their position within the dunefield depositional environment. The values established using this technique allow characterisation of the subsurface in areas with relative paucity of outcrop. Added to this is the understanding that facies type affects both diagenesis (e.g., Bouch et al., 2006) and deformation (Beach et al., 1997). Therefore, the prediction of facies distribution and properties in the subsurface allows provision of data for the construction of flow models that depict flow in units at the sub-seismic scale. These models are especially useful when characterising flow in the Sherwood Sandstone Group which is relevant to understanding contaminant flow models (Tellam and Barker, 2006) and understanding the potential impact on the use of the Sherwood Sandstone Group as a CCS site (Williams et al., 2013). This sub-seismic scale modelling is also beneficial in estimating storage capacity in potential low-temperature geothermal systems, and understanding the results of injection-production tests in these strata. Likely flow-paths of groundwaters of varying salinities can be predicted and how these may evolve with time which impacts on the design of low-temperature geothermal systems.
The continuation of this study will comprise the addition of outcrop data to both extend and test the calculated vertical versus lateral extent ratios. The data will be analysed statistically to ensure a robust dataset from which the ratios are derived. The ratios will then be applied to additional existing core data and new core data with the completion of drilling at the Cheshire Energy Research Field Site. Further investigation will be made into the significance of variation in interdune thickness and the relevance to sedimentation rates, with thicker dry interdunes suggesting slower deposition rates and therefore a dry system. This and other spatial data from outcrop and borehole core data associated with this project could form the basis of future statistical modelling approaches whereby static 3-D geological models are attributed with representative porosity and permeability values for each different facies or facies associations. Recent numerical modelling studies (e.g. Newell and Shariatipour, 2016) have used cell sizes that are too large to account for the heterogeneities identified in this study; however with future improvements in computing capabilities, it will be important to model these sub-seismic scale heterogeneities to fully understand the impact they have on flow in these units.
Core material used in this study is available from the National Geosciences Data Centre, British Geological Survey, Nottingham NG12 5GG.
JT and DP collected core data and completed the desk study. All authors wrote the article. JT finalized the manuscript. All authors read and approved the final manuscript.
The authors declare that they have no conflict of interest.
This article is part of the special issue “European Geosciences Union General Assembly 2019, EGU Division Energy, Resources & Environment (ERE)”. It is a result of the EGU General Assembly 2019, Vienna, Austria, 7–12 April 2019.
Staff at the National Geosciences Data Centre, particularly Tracey Gallagher and Scott Renshaw, are thanked for facilitating access to core materials used in this study. Richard Haslam is thanked for discussions that improved the manuscript. The authors publish with the approval of the Executive Director, British Geological Survey, Natural Environment Research Council.
This paper was edited by Christopher Juhlin and reviewed by Christopher Juhlin and one anonymous referee.
Beach, A., Lawson Brown, J., Welbon, A. I., McCallum, J. E., Brockbank, P., and Knott, S.: Characteristics of fault zones in sandstones from NW England: application to fault transmissibility, Geol. Soc. Spec. Publ., 124, 315–324, 1997.
Bloomfield, J. P., Moreau, M. F., and Newell, A. J.: Characterization of permeability distributions in six lithofacies from the Helsby and Wilmslow sandstone formations of the Cheshire Basin, UK, in: Fluid flow and solute movement in sandstones: the onshore UK Permo-Triassic red bed sequence, edited by: Barker, R. D. and Tellam, J. H., Geol. Soc. Spec. Publ., 263, 83–101, 2006.
Bouch, J. E., Hough, E., Kemp, S. J., McKervey, J. A., Williams, G. M., and Greswell, R. B.: Sedimentary and diagenetic environments of the Wildmoor Sandstone Formation (UK): implications for groundwater and contaminant transport, and sand production, Geol. Soc. Spec. Publ., 263, 129–153, https://doi.org/10.1144/GSL.SP.2006.263.01.07, 2006.
Bridge, D. and Hough, E.: Geology of the Wolverhampton district, Sheet description of the British Geological Survey Sheet 153 (England and Wales), ISBN 085272 3997, 2002.
Crofts, R. G., Hough, E., Humpage, A. J., and Reeves, H. J.: Geology of the Manchester district – a brief explanation of the geological map, Sheet Explanation of the British Geological Survey, 1 :50 000 Sheet 85 Manchester (England and Wales), 2012.
Henares, S., Caracciolo, L., Cultrone, G., Fernández, J., and Viseras, C.: The role of diagenesis and depositional facies on pore system evolution in a Triassic outcrop analogue (SE Spain), Mar. Petrol. Geol., 51, 136–151, https://doi.org/10.1016/j.marpetgeo.2013.12.004, 2014.
Hough, E., Pearce, J. M., Kemp, S. J., and Williams, G. M.: An investigation of some sediment filled fractures within redbed sandstones of the UK, P. Yorks. Geol. Soc., 56, 43–55, 2006.
Howard, A. S., Hough, E., Crofts, R. G., Reeves, H. J., and Evans, D. J.: Geology of the Liverpool district – a brief explanation of the geological map, Sheet Explanation of the British Geological Survey, 1 :50 000 Sheet 96 Liverpool (England and Wales), 2007.
McKie, T. and Williams, B.: Triassic palaeogeography and fluvial dispersal across the northwest European Basins, Geol. J., 44, 711–741, https://doi.org/10.1002/gj.1201, 2009.
Marriott, S. B. and Hillier, R. D.: Fluvial style in the Lower Old Red Sandstone: examples from southwest Wales, UK, P. Geologist. Assoc., 125, 534–547, 2014.
Medici, G., West, L. J., and Mountney, N. P.: Characterizing flow pathways in a sandstone aquifer: Tectonic vs. sedimentary heterogeneities, J. Contam. Hydrol., 194, 36–58, 2016.
Mountney, N. P. and Thompson, D. B.: Stratigraphic evolution and preservation of aeolian dune and damp/wet interdune strata: an example from the Triassic Helsby Sandstone Formation, Cheshire Basin, UK, Sedimentology, 49, 805–833, 2002.
Mountney, N. P.: Eolian facies models, in: Facies Models Revisited, edited by: Posamentier, H. W. and Walker, R. G., Soc. Econ. Pa., 84, 19–83, 2006.
Newell, A. J. and Shariatipour, S. M.: Linking outcrop analogue with flow simulation to reduce uncertainty in sub-surface carbon capture and storage: an example from the Sherwood Sandstone Group of the Wessex Basin, UK, in: The value of outcrop studies in reducing subsurface uncertainty and risk in hydrocarbon exploration and production, edited by: Bowman, M. and Jordan, C. J., Geol. Soc. Spec. Publ., 436, 231–246, 2016.
Steel, R. J. and Thompson, D. B.: Structures and textures in Triassic braided stream conglomerates (“Bunter” Pebble Beds) in the Sherwood Sandstone Group, north Staffordshire, England, Sedimentology, 30, 341–367, 1983.
Tellam, J. H. and Barker, R. D.: Towards prediction of saturated-zone pollutant movement in groundwaters in fractured permeable-matrix aquifers: the case of the UK Permo-Triassic sandstones, Geol. Soc. Spec. Publ., 263, 1–48, https://doi.org/10.1144/GSL.SP.2006.263.01.0, 2006.
Thompson, D. B.: Sedimentation of the Triassic (Scythian) red pebbly sandstones in the Cheshire Basin and its margins, Geol. J., 7, 183–261, 1970.
Wakefield, O. J. W., Hough, E., and Peatfield, A. W.: Architectural analysis of a Triassic fluvial system: The Sherwood Sandstone of the East Midlands Shelf, UK, Sediment. Geol., 327, 1–13, https://doi.org/10.1016/j.sedgeo.2015.07.006, 2015.
Wealthall, G. P., Steele, A., Bloomfield, J. P., Moss, R. H., and Lerner, D. N.: Sediment-filled fractures in the Permo-Triassic sandstones of the Cheshire Basin: observations and implications for pollutant transport, J. Contam. Hydrol., 50, 41–51, 2001.
Williams, J. D. O., Jin, M., Bentham, M., Pickup, G. E., Hannis, S. D., and Mackay, E. J.: Modelling carbon dioxide storage within Closed structures in the UK Bunter Sandstone Formation, Int. J. Greenh. Gas Con., 18, 38–50, 2013.