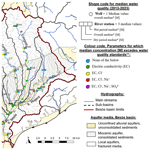
Where could surface water be used for Managed Aquifer Recharge (MAR) in the Besòs catchment, considering quantity and quality?
Luciana Scrinzi
Sandra Pérez
Estanislao Pujades
Enhancing aquifer recharge could support climate adaptation in the Besòs basin and in the rest of Catalonia (Spain). This study examines how water quality in aquifers and streams varies along the catchment, and changes in surface water quality during wet and dry periods. Data from 2013 to 2023 from 20 river stations and 26 wells were used to map salinity (EC, Na+, Cl−, SO) and nutrient parameters (PO, NH4+, NO, TOC). Results indicate declining water quality downstream, necessitating salinity control during dry periods and ongoing nutrient management even in wet periods to preserve groundwater quality. These findings will inform the development of numerical models to test MAR methods and recharge strategies in the basin.
- Article
(1906 KB) - Full-text XML
- BibTeX
- EndNote
With increasing floods and droughts with climate change, groundwater is key for adaptation due to higher climatic resilience than surface water (Cuthbert et al., 2019). However, overusing groundwater can deplete aquifers, especially during droughts (Taylor et al., 2013). Managed Aquifer Recharge (MAR), the purposeful recharge of aquifers for later recovery or environmental benefit, is gaining attention as a climate adaptation measure (Zheng et al., 2021). Suitable aquifers are needed for effective MAR, as well as adequate land and sufficient quantity and quality of recharge sources (Alley et al., 2022). Rivers and streams are often the main water sources for MAR (Sprenger et al., 2017). In “Cuencas Internas de Catalunya” (CIC), some sub-catchments already face irregular surface water availability and quality issues (ACA, 2023). This study aims to identify spatial variations in water quality in the Besòs basin (a CIC sub-catchment) during wet and dry periods to inform mapping of MAR feasibility for climate adaptation.
Open databases (Visor ACA, 2024; ACA SDIM, 2024; Geoportal, 2024; ICGC GT-V Hydrogeology maps, 2024) were used to map the river network and aquifers in the Besòs basin for MAR. Groundwater and river hydrochemistry data from 2013 to 2023 were gathered from ACA-SDIM. This period and selected monitoring stations (26 wells of median depth 15 m, yearly monitoring; 20 river stations, near-monthly monitoring) provided the most complete data. Variables selected were EC, Na+, Cl−, SO (salinity), and PO, NH4+, NO, TOC (nutrients), relevant for MAR risk-based management (Dillon et al., 2009a) and assessed against CIC thresholds (ACA, 2023). Daily river flow time series (2007–2023) at the Santa Coloma de Gramanet gauge station (SCG) were analysed. Three median concentrations (2013–2023) were calculated for each water chemistry variable in river stations: (1) overall median (all measurements 2013–2023); (2) dry periods median (measurements when Q (SCG) < 2 m3 s−1); (3) wet periods median (measurements when Q (SCG) > 5 m3 s−1). A single overall median value (1) was calculated for each well.
River flow time series near the basin's outlet points to an increasing frequency of periods with flow below P25 during two or more consecutive days (Fig. 1a). From 2021–2023, these low-flow periods added up to an average of 204 days a year with flows below 2 m3 s−1, aligning with global climate projections of decreased mean rainfall and more frequent extreme events (Zittis et al., 2021). In contrast, wetter years (like 2018–2020) have more periods of continued high flow (above P75).
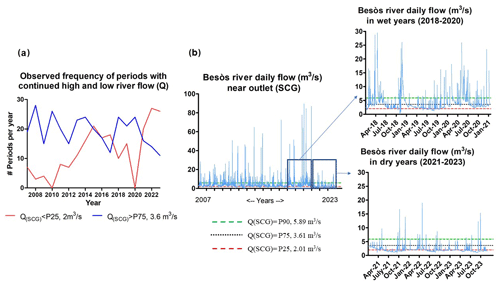
Figure 1(a) Frequency of observed periods with continued high and low river flow (below P25 and above P75 for more than 1 d, respectively) at SCG. (b) Observed River Flow at SCG in 2007–2023, as well as during wet and dry years. With data from ACA SDIM. Note: in 2018–2020 graph, data points outside Y axis range are not shown.
Peak flow events (above P90, 5.89 m3 s−1) may also become less frequent in dry years (Fig. 1b). Between 2007–2023, there were an average of 36 peak flow days annually. In contrast, from 2021–2023 there were only 7 peak flow days annually, which could affect natural diffuse recharge, indirect recharge through surface water, and river water quality (Healy, 2010). The latter is assessed in Figs. 2 and 3, contrasting how salinity and nutrients concentrations in streams across the basin change in times of high and low flow, potentially affecting their suitability as water source for MAR. Regarding hydrogeology, alluvial aquifers in the basin (Fig. 2) may be best for surface spreading methods in MAR systems, as they are unconfined and have higher permeability (Bouwer, 2002). Due to their hydraulic connection to alluvial aquifers and bigger thickness (ICGC), the rest of the aquifers in the basin should also be considered in future studies even though they may hold moderate interest for MAR due to a lower permeability.
Although soils and aquifers can mitigate pollution during infiltration and storage respectively, groundwater quality must be ensured in sustainable MAR, including for nutrients and salinity (Dillon et al., 2009a). Results show that overall median EC in Besòs alluvial aquifers was 1314 µS cm−1, with all wells meeting groundwater standards (≤ 2000 µS cm−1) and 77 % below 1500 µS cm−1. River stations had a median EC of 1200 µS cm−1, with 75 % below 1500 µS cm−1.
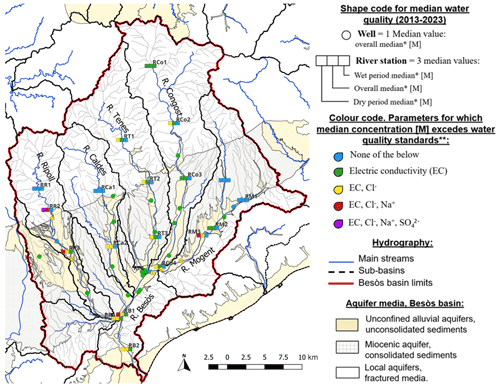
Figure 2Monitoring points median concentration [M] classed with relation to water quality standards for salinity variables. ∗ see Methodology section. For surface water bodies in Besòs (ACA, 2023): < 1000 us cm−1 for EC, < 200 mg L−1 for Na, Cl and SO4.
Some stations (RR2, RR3, RM3) exceeded EC and Cl standards during average and dry periods with, additionally, Na concentration above standards in dry periods. Nearby wells show high EC and Cl levels. Other stretches (RCo2, RCo4, RT1, RT2, RT3) had Cl above standards only in dry periods. During wet periods, all river stations have median EC below 1200 µS cm−1 and Na and Cl below 200 mg L−1. On the other hand, nitrate is the primary pollutant in aquifers (Fig. 3), with a median concentration of 39 mg L−1; 69 % of wells exceed 25 mg L−1, and 46 % surpass the 50 mg L−1 groundwater limit. In contrast, river stations have an overall median of nitrate of 16.9 mg L−1, with 35 % above drinking water standards, notably in lower Ripoll, lower Caldes, intermediate Tenes, upper Congost, and upper Mogent. Unlike with salinity, there is little variation in nitrate concentrations between wet and dry periods.
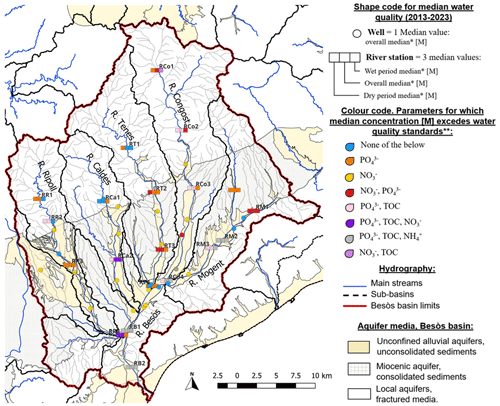
Figure 3Monitoring points median concentration [M] classed with relation to water quality standards for nutrient variables. ∗ see Methodology section. For surface water bodies in Besòs basin (ACA, 2023): < 0.5 mg L−1 for PO4 and NH4, < 5 mg L−1 for TOC, < 25 mg L−1 for NO3.
Finally, phosphate is prevalent in rivers, with overall median above 0.5 mg L−1 standard in 85 % of stations. Most tributaries except Tenes exceed the TOC standard as well (5 mg L−1) in lower stretches. Lower Mogent, Congost, and Besòs stations also have NH4 levels above 0.5 mg L−1. In contrast, aquifers have a median PO4 concentration of 0.1 mg L−1, with only one well exceeding standards for PO4, one for NH4 (both near RCo4) and no wells surpassing TOC standards. All upper stretches show the best water quality regarding nutrient load. Main anthropic pressures in aquifers, which may affect salinity and nutrients, are occupation of soil by industrial and urban areas including sewage leaks, followed by non-treated waste from animal production facilities and industries. Wastewater treatment plants and untreated industrial waste impact rivers, as well as agricultural and urban land uses in the catchment. Agricultural practices contribute pollutants such as fertilizers and pesticides to both aquifers and rivers (ACA, 2020). Especially in heavily polluted stretches, pre-treatment methods may be needed to address water quality issues during MAR, complementing natural attenuation processes. This could include the use of wetlands, microfiltration, granular activated carbon, reactive barriers and SMART methods (Dillon et al., 2009b; Hellauer et al., 2018; Valhondo et al., 2020).
The best water quality for MAR may be found along the Tenes, with a need to control sources of PO4 and NO3 affecting RT2 and RT3, and to address sources that influence salinity during dry periods. The Ripoll, Caldes, and Mogent show marked decreases in water quality at RR2, RCa2, and RM2, respectively, indicating pollution sources that increase nutrients and salinity (the latter, worsened during dry periods), affecting potential suitability for MAR. In the Congost basin, salinity-related pollution downstream of RCo2 improves during wetter periods, but not nutrients. For effective pollution control, integrated approaches may be needed to engage land users in MAR for climate adaptation (Piacentini and Rossetto, 2020). These results will inform the development of flow and transport models with MAR scenarios in the Besòs catchment, further exploring interactions between climate, rivers and underlying aquifers. Future assessments of MAR feasibility and selection of MAR methods will consider jointly water quality and quantity in the main tributaries, as well as aquifers characteristics including natural attenuation capacity. Further efforts will go into understanding pollution sources driving salinity and nutrient loads in the basin, as well as their effect on contaminants of emerging concern (CECs) (Geissen et al., 2015) in aquifers and rivers.
All underlying research data can be accessed from public databases. River flow and water chemistry data can be downloaded from the database for water quantity and quality control of the Agencia Catalana de l'Aigua (ACA SDIM). GIS layers can be downloaded from Visor ACA and Geoportal.
LS contributed with data collection, data processing, formal analysis and original draft preparation. EP contributed with conceptualization and supervision. SP provided critical review, commentary and revision.
The contact author has declared that none of the authors has any competing interests.
Publisher’s note: Copernicus Publications remains neutral with regard to jurisdictional claims made in the text, published maps, institutional affiliations, or any other geographical representation in this paper. While Copernicus Publications makes every effort to include appropriate place names, the final responsibility lies with the authors.
This article is part of the special issue “Groundwater management in the context of global change: integrating innovative approaches (EGU2024 HS8.2.1 session)”. It is a result of the EGU General Assembly 2024, Vienna, Austria, 14–19 April 2024.
The doctoral research receives the support of a fellowship from the ”la Caixa” Foundation (ID 100010434), fellowship code “B006133”. Also: CEX2018-000794-S funded by MCIU/AEI/10.13039/501100011033. MICIU/AEI/10.13039/501100011033 and European Union NextGenerationEU/PRTR through the grant CNS2023-144051. LS and EP acknowledge the support from MICIU/AEI/10.13039/501100011033 and European Union NextGenerationEU/PRTR through the grant CNS2023-144051 and MICIU/AEI /10.13039/501100011033 and European Union through the grant PCI2024-153452 (this grant belongs to the WATER4MED project funded by the PRIMA programme in 2023).
This research has been supported by the “la Caixa” Foundation (grant no. B006133). This paper has been partially supported by the PRIMA Programme and the European Union through the WATER4MED Project. Authors acknowledge the support from MCIU/AEI/10.13039/501100011033 (grant no. CNS2023-144051, cofounded by the European Union NextGenerationEU/PRTR; grant no. PCI2024-153452, cofounded by the European Union; grant no. CEX2018-000794-S).
The article processing charges for this open-access publication were covered by the CSIC Open Access Publication Support Initiative through its Unit of Information Resources for Research (URICI).
This paper was edited by Anna Jurado Elices and reviewed by two anonymous referees.
ACA: Document IMPRESS 2019. Estudi general de la demarcació, anàlisi d’impactes i pressions de l’activitat humana, i anàlisi econòmica de l’ús de l'aigua a les masses d’aigua al Districte de conca fluvial de Catalunya, 245 pp. , 2020.
ACA: Pla de gestió del districte de conca fluvial de Catalunya 2022–2027, 553 pp., 2023.
Alley, W., Dillon, P., and Zheng, Y.: Basic Concepts of Managed Aquifer Recharge, Managed Aquifer Recharge: Overview and Governance, ISBN 978-1-3999-2814-4, 90 pp., 2022.
ACA SDIM: Online database for water quantity and quality control of the Agencia Catalana de l'Aigua (ACA), https://aplicacions.aca.gencat.cat/sdim21/ (last access: 15 March 2024), 2024.
Bouwer, H.: Artificial recharge of groundwater: hydrogeology and engineering, Hydrogeol. J., 10, 121–142, https://doi.org/10.1007/s10040-001-0182-4, 2002.
Cuthbert, M. O., Gleeson, T., Moosdorf, N., Befus, K. M., Schneider, A., Hartmann, J., and Lehner, B.: Global patterns and dynamics of climate–groundwater interactions, Nat. Clim. Change, 9, 137–141, https://doi.org/10.1038/s41558-018-0386-4, 2019.
Dillon, P., Declan, P., Pavelic, P., Toze, S., Vanderzalm, J., Levett, K., Stevens, D., and Newland, P.: Australian Guidelines for Water Recycling. Managed Aquifer Recharge, Australia NRMMC, ISBN 1921173475, 237 pp., 2009a.
Dillon, P., Pavelic, P., Page, D., Beringen, H., and Ward, J.: Managed aquifer recharge: An introduction, NationalWater Commission Waterlines Report Series, 13, ISBN: 978-1-921107-71-9, 64 pp., 2009b.
Geissen, V., Mol, H., Klumpp, E., Umlauf, G., Nadal, M., Van Der Ploeg, M., Van De Zee, S. E. A. T. M., and Ritsema, C. J.: Emerging pollutants in the environment: A challenge for water resource management, Int. Soil Water Conserv. Res., 3, 57–65, https://doi.org/10.1016/j.iswcr.2015.03.002, 2015.
Geoportal: Online platform for visualization, access and analysis of geographic information by Spanish authorities (Ministerio de Agricultura, Pesca y Alimentación; Ministerio para la Transición Ecològica y el Reto Demográfico), https://sig.mapama.gob.es/geoportal/ (last access: 15 March 2024), 2024.
Healy, R. W.: Estimating Groundwater Recharge, Cambridge university press, ISBN 978-1-139-49139-6, 245 pp., 2010.
Hellauer, K., Karakurt, S., Sperlich, A., Burke, V., Massmann, G., Hübner, U., and Drewes, J. E.: Establishing sequential managed aquifer recharge technology (SMART) for enhanced removal of trace organic chemicals: Experiences from field studies in Berlin, Germany, J. Hydrol., 563, 1161–1168, https://doi.org/10.1016/j.jhydrol.2017.09.044, 2018.
ICGC GT-V Mapa Hidrogeológico: Catalogue of Hydrogeological Maps at a 1:25 000 Scale for Catalunya by Institut Cartogràfic i Geològic de Catalunya (ICGC). https://www.icgc.cat/es/Datos-y-productos/Geoinformacio-geologica/Cartografia-hidrogeologica/GT-V-Mapa-hidrogeologico-125000 (last access: 15 March 2024), 2024.
Piacentini, S. M. and Rossetto, R.: Attitude and Actual Behaviour towards Water-Related Green Infrastructures and Sustainable Drainage Systems in Four North-Western Mediterranean Regions of Italy and France, Water, 12, 1474, https://doi.org/10.3390/w12051474, 2020.
Sprenger, C., Hartog, N., Hernández, M., Vilanova, E., Grützmacher, G., Scheibler, F., and Hannappel, S.: Inventory of managed aquifer recharge sites in Europe: historical development, current situation and perspectives, Hydrogeol. J., 25, 1909–1922, https://doi.org/10.1007/s10040-017-1554-8, 2017.
Taylor, R. G., Scanlon, B., Döll, P., Rodell, M., Van Beek, R., Wada, Y., Longuevergne, L., Leblanc, M., Famiglietti, J. S., Edmunds, M., Konikow, L., Green, T. R., Chen, J., Taniguchi, M., Bierkens, M. F. P., MacDonald, A., Fan, Y., Maxwell, R. M., Yechieli, Y., Gurdak, J. J., Allen, D. M., Shamsudduha, M., Hiscock, K., Yeh, P. J.-F., Holman, I., and Treidel, H.: Ground water and climate change, Nat. Clim. Change, 3, 322–329, https://doi.org/10.1038/nclimate1744, 2013.
Valhondo, C., Carrera, J., Martínez-Landa, L., Wang, J., Amalfitano, S., Levantesi, C., and Diaz-Cruz, M. S.: Reactive Barriers for Renaturalization of Reclaimed Water during Soil Aquifer Treatment, Water, 12, 1012, https://doi.org/10.3390/w12041012, 2020.
Visor ACA: Cartographic Viewer by Agencia Catalana de l'Aigua (ACA) with information layers on the water environment in Catalonia, https://sig.gencat.cat/visors/VISOR_ACA.html (last access: 15 March 2024), 2024.
Zheng, Y., Ross, A., Villholth, K. G., and Dillon, P.: Managing aquifer recharge: a showcase for resilience and sustainability, UNESCO, Paris, Retrieved 10 August 2021, ISBN 978-92-3-100488-9, 379 pp., 2021.
Zittis, G., Bruggeman, A., and Lelieveld, J.: Revisiting future extreme precipitation trends in the Mediterranean, Weather Clim. Extrem., 34, 100380, https://doi.org/10.1016/j.wace.2021.100380, 2021.